Question 42: What water sources are you using for desalter waterwash, and what are the advantages and disadvantages of each? What role does desalter waterwash source and injection location play in desalter performance?
SOLOMON (Athlon Solutions)
As Gordon said, these were throwback questions that Chris McDowell answered a while ago. It would have been great to see those actual answers. I am sure they are very similar to what we have now; but still, just to see how much the desalting process may or may not have changed.
In the years that some of my other colleagues and I have been working on desalter waterwash practices, we have some Best Practices we think work well with all varieties of crude. First is wash water. Yes, wash water. More wash water: whatever you can get. Typically, 5 to 8% of the crude charge is what we recommend. Heavy crudes may be even a little higher, if we can get it.
Wash water quality is also another very important aspect. We look for wash water somewhere between 6 and 8, in terms of pH, which contains less than 50 ppm of ammonia; is low in chlorides; and, has low hardness, low oil content, and – obviously – low oxygen content.
Preferred sources: There are a variety of sources we like to see. Stripped sour water is usually our best source. Sour water from the overhead systems, such as the vacuum overhead, is very good, too, as are non-scaling freshwater and steam condensate. Steam condensate is obviously an expensive alternative, but it can be used.
Over the years, we have experimented in a technical sense and looked at different ways of injecting wash water. What we found, at least at Athlon, is that we try to get as much of the wash water upstream as possible. This is actually upstream of the crude charge pump. We want to get some very good mixing and improve our contact time. After that, just a little ahead of the crude preheat is the next best source. Finally, at the mix valve where we typically see a problem is that when we inject water and chemical at the mix valve, and we just do not have a lot of contact time. I cannot tell you how many operations I have seen where, well, the mix valve really is not the mix valve. It is a control valve or something else; or it is wide open. So, inject wash water as far upstream as possible.
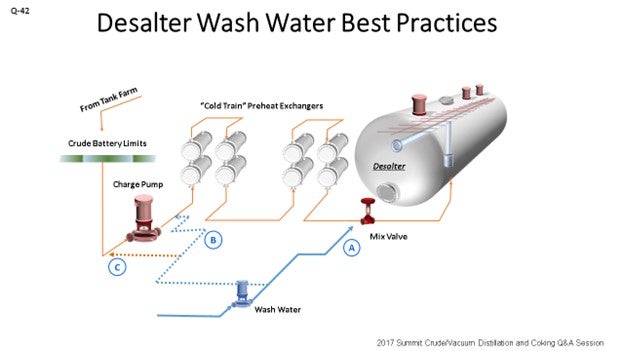
There are some advantages of the different sources of overhead water. Vacuum overhead tends to be fairly light in hydrocarbons and low in pH, but there are some disadvantages as well. It can result in recycling of any tramp amines that are in the system, so we want to be careful of that.
These are some pictures of basically what is going on, in terms of crude oil and then desalted crude. In the picture on the very left, we see raw crude – just filtered with toluene and not washed with water – containing about 85.5 ptb (pounds per 1,000 barrels) of salt. You can see those crystalline forms. The top right photo shows what happens when we do it just ahead of the desalter. At that point, you still start to see some good work. We are getting most of the salts out, but still about 3.5 ptb remains there. Finally, what we have seen when we move it into the suction of the charge pump is that, again, we have 0.9 ptb. We are contacting a lot of the salts and really doing a good job removing them in the water.
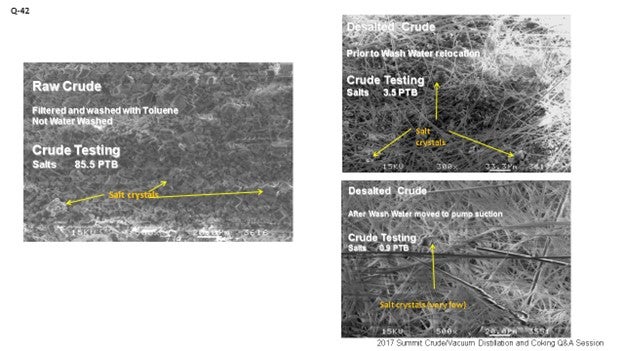
Some people might say, “Well, are we creating a very stable emulsion by doing that?” That is a possibility; it can happen. But crude charge pumps tend to be good devices for moving fluid and not necessarily very high shear mixing apparatus; so, most of the time, we are seeing very good results from this operation.
Sometimes we have talked about water that is soluble in the oil. When that happens, though, just the water goes into the oil. The salts stay behind and end up in this more crystalline form. We want to be careful that we are not getting a lot of the soluble water out of the crude and just leaving salts behind; because by that time, we have already lost the opportunity to contact the salt and get it out of the system. That is why we try to continue to move it as far upstream of the water injection as possible. The pictograph below shows the solid salts that can be left behind in the crude oil.
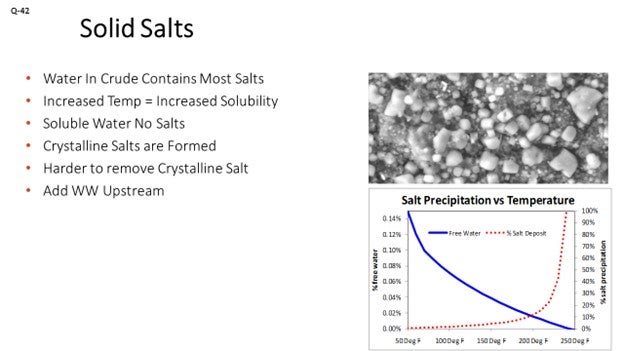
So, those are basically the Best Practices. I do not think they have changed in a long time, since Chris gave the response several years ago. It would have been great to have some of the throwback response, but perhaps the next time.
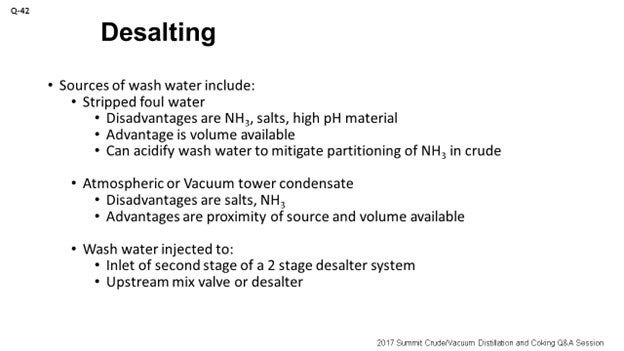
LÉGARÉ (Andeavor Martinez Refinery)
I do not really have much to add after that discussion. I will say, though, that is there is a difference between what is ideal – as far as makeup wash water is concerned – and what you actually get in a refinery. So, it is probably best to put some thought into how you are going to mitigate your deficiencies in your gaps, as far as pH and ammonia content are concerned. One of the techniques we have looked at in the past is acidifying the wash water to deal with high amounts of ammonia. That technique has been effective for us in the past.
Hydraulic limits: If you are trying to get your stripped foul water over to a crude unit and the sources are not close by, then those hydraulic limits can also limit the amount of makeup you have at the desalters. It becomes a juggling act between what you have and what you would like to have. Then, you have to come up with the proper justification to put in these new projects or new facilities to move forward to get closer to Best Practice.
BILL CATES (Hunt Refining Company)
One subject the panelists did not discuss, which is one of our considerations, is wash water percentage. One of the ways to get it up is to do recycle off the bottom of the desalter. There are pros and cons to that method; because obviously, the water is hot. The other fact is that it actually contains some salt; meaning, it has a heavier density, which helps the water get it back out. Very seldom are you super-saturated with that amount of salt. So, do you have any comments about using recycle to supplement wash water in front of the mix valve?
SOLOMON (Athlon Solutions)
Just like Eric said, usually what you have to use is what you can get. Certainly, we have seen recycled wash water. Of course, we have a two-stage of desalting that is always occurring. Yes, you just have to be careful. Use the best quality. But often, as Eric mentioned, you just use what you have there.
KEVIN SOLOMON (Athlon Solutions)
Wash Water Best Practices
Over the years, we have established several Best Practices concerning desalter wash water rates, quality, injection locations, and sources that – when utilized – help ensure optimum crude oil desalting.
We have found that wash water rates of 5 to 8% of crude charge is sufficient for light- to mid-API crudes and that heavier crude need slightly higher rates. Wash water quality is equally as important as wash water rates. Ideal wash water specifications are:
-
pH level of 6 to 8,
-
Contain less than 50 ppm ammonia,
-
Contain less than 50 ppm chlorides, and
-
Have low hardness, low oil content, and low oxygen content.
Our preferred wash water sources are stripped sour water, sour water from overhead systems (such as vacuum overhead condensate), and non-scaling fresh water and steam condensate. The advantages of overhead water are:
-
It removes light hydrocarbons from the vacuum overhead water (less hydrocarbons to sour water stripper), and
-
The lower pH helps lower total wash water pH, especially if there is a higher pH wash water source in use.
The disadvantages of atmospheric or vacuum overhead water are that there is the possibility of recycling tramp amines back into crude oil.
The location of wash water injection is also important. Our Best Practice is to inject wash water far upstream of the desalters to provide sufficient time to contact any water (and thereby salt) in the crude oil. Our preferred injection points (in order of preference) are:
-
At the suction to the crude charge pump,
-
Ahead of the pretreat train, and
-
Ahead of the mix valve.
There are several advantages to injecting wash water ahead of the crude charge pump. First is the improved contact of wash water with crude oil, thereby improving desalting. Injecting ahead of the preheat helps to heat up desalter wash water, which minimizes formation of crystalline salts in cold preheat train and therefore results in less fouling and higher desalter temperature. This injection location can permit running desalter mix valve lower with equal or better desalter salt removal; hence, lower desalter pressure which – in some instances – can lead to slightly higher crude throughput.
There are some disadvantages of injecting wash water upstream of crude charge pump. It is possible that the increased shear stress resulting from the pump can create a tighter emulsion in some cases. Without the right EB and low oil/water residence time, this emulsion may be hard to resolve. If the desalter wash water is coming from the second stage, it will be 250°F, which will then be injected into the cold crude. On a single-stage, the brine water exchanger never gets the feed water up to desalter temperature; so, it is not as bad a problem. Using cold, fresh water as part of the charge pump wash water can help, even if only a portion of the total water is going to the pump suction.
ERIC LÉGARÉ (Andeavor Martinez Refinery)
Stripped sour water is commonly used as makeup water to a desalter. Disadvantages of stripped foul water include NH3 in the wash water as it can cycle up and increase the risk of chloride salts in your tower/overhead system at high pH levels. Acidifying wash water with high NH3 content can be a solution to reduce the partitioning of NH3 into the crude phase. The advantage of this water source are the high volumes available at low cost as this stream is normally routed to the wastewater plant.
Alternatives would be condensate from the atmospheric or vacuum fractionator overhead systems. The advantages of this stream are both its availability and proximity to the desalter. The disadvantage would be the chloride salt content in this water stream, if there was no supplemental waterwash in this system, pH, and the increased potential for amines.
Injection of wash water goes to the second stage of a two-stage system. Alternately, some or all of the water can also be injected upstream the mix valve to maximize mixing with the crude upstream of the desalters.
CHRIS CLAESEN (NALCO Champion)
The best desalter wash water sources are stripped sour water with low salt and solids content or BFW, but this material is expensive. Try to avoid waters containing oxygen, hardness, tramp amine, and high salt levels. Water injection location has a significant impact when very low salt levels are required. Injecting part of the water in the cold preheat will help remove some of the crystalline salt and prevent drying out of the crude. The amount of water that needs to be injected at each specific location will depend on the unit design and the feed properties.
DENNIS HAYNES (NALCO Champion)
The list of potential desalter wash water sources is long. An important factor to consider when choosing a source is to review which contaminants are in the water. Oxygenated sources, such as industrial water, may cause an increase in SOx to the distillation column. Stripped sour water is a common source and has been used effectively in many cases; yet, caution is needed when the pH is high, especially if the pH is due to caustic addition at the SWS. Atmospheric column overhead water may or may not be acceptable, depending on the amine content and the pH of the desalter wash water blend. Vacuum overhead hotwell water is typically a good source; yet it needs to be monitored to make sure it does not bring a large amount of emulsified hydrocarbon condensate to the desalter. Moderate to slightly lower pH wash water typically results in better emulsion resolution and contaminant removal. Injection of wash water to points that facilitate good yet controlled mixing will also result in better contaminant removal. Each system needs to be optimized based on feed quality and characteristics and system mechanical design characteristics.
PHIL THORNTHWAITE (NALCO Champion)
The provision of adequate, good quality desalter wash water can present a significant challenge to some refineries; some sources of water are good, and some not so good. Utilizing poor quality wash water can result in processing issues, contributing to desalter upsets, corrosion, fouling, and wastewater treatment plant issues. So, the choice of the water source is critical to good desalter performance. Ultimately, the best way to identify whether a water source can be added to the desalter wash water is to analyze the contaminants that are present in that particular source and in the overall wash water charge, followed by an assessment of the risk that these incur.
The best water sources, and the ones more commonly utilized, are overhead sour waters from the crude and vacuum units and good quality stripped sour water. There are considerations, though, in that the amine content of the overhead sour waters, whether it be from neutralizers or tramp amines, should not present an amine recycle risk that could increase the risks of salt formation. Likewise, the amine content of the VDU sour water should be considered, as well as well the level of hydrocarbons in this stream, as this can potentially lead to desalter upsets and/or impact on desalter effluent quality. If stripped sour waters are used, ideally, they should be low in ammonia and also phenols, while bottoms from an SWS that injects caustic to increase ammonia stripping is best avoided. High ammonia levels can elevate the pH of the water that can increase emulsion formation; and depending on the pH, it will readily partition into the oil phase, passing to the crude unit overheads where it can increase the risk of salt formation. Phenols can impact on effluent water quality and have a huge detrimental impact on the secondary processes of the wastewater treatment plant, while they can also contribute to emulsion formation and stabilization.
For those refiners limited in the amount of wash water available, a brine recycle can be an effective means of boosting available wash water and improving desalter performance. However, care has to be taken when utilizing desalter brine in this way; if there is a deterioration in effluent water quality, recycling this stream can precipitate a much larger desalter upset.
With respect to wash water injection locations, it is typical to inject a portion of the total wash water in front of the cold preheat exchangers with the remainder just before the mix valve. The portion injected before the cold preheat dilutes the scaling salts present in the crude, reducing the risk of exchanger fouling. However, varying the ratio of water injected at these two locations can improve salt removal since the cold preheat exchangers can help improve mixing with dispersed brine droplets and potentially crystalline salts. This ratio can be easily determined through a desalter optimization study using the correct analytical methodology.
GLEN SCATTERGOOD (NALCO Champion)
We use primarily stripped sour water along with vacuum overhead condensate. Ammonia content of the stripped sour water must be controlled in order to manage the combined wash water pH. An elevated wash water pH is caused by increased ammonia in the stripped sour water, which lead to degraded dehydration of the desalted crude inside the desalter vessel.
We use stripped sour water as the source for desalter wash water. A brine recycle is in place to increase the total wash water volume greater than 7% based on crude charge. The addition of the recycle and increase in total wash water has reduced atmospheric distillation tower overhead chlorides by 20%.
SAM LORDO (NALCO Champion)
The typical desalter wash water sources are:
-
Stripped sour water and
-
Condensates.
The typical desalter wash water quality is:
pH |
6-8 |
NH3 |
< 10 ppm |
Cl- |
< 25 ppm |
Temperature |
> 180°F (82°C) |
Oil |
Nil |
Oxygen |
ppb (parts per billion) |
Stripped sour water tends to run high in pH and ammonia. The high pH increases the potential to stabilize emulsions. It is also possible that the stripped sour water could have other contaminates present, like cyanide, organic acids, and amines (tramp and internally generated).
Condensates from the CDU tend to be better to use overall; however, they, too, can contain amines (from neutralizers being applied and from crude oil sources, organic acids, H2S, ammonia, etc.) Condensates from other units – like delayed cokers, fluid catalytic crackers (FCCs), hydroprocessing unit stabilizer/stripper towers, etc. – can introduce other contaminates not normally found in the CDU or they can increase the concentration of other contaminates. For example, an FCC condensate can easily have more than 2,000 to 4,000 ppm ammonia present, compared to a typical CDU overhead of 40 to 100 ppm.
Solids, high pH, and other contaminates present in the wash water can result in the stabilization of desalter emulsions or can change the solubility of contaminates in crude oil, which could result in unwanted contaminates going out of the desalter to the downstream equipment.
Question 70: Electrostatic precipitator (ESP) fines handling is often complicated by fluidization and mechanical integrity issues. How often do you experience these types of fines handling issues, and what are some of your Best Practices to successfully mitigate these issues? What are your Best Practices for safe fines withdrawal from the ESP?
FOOTE (CHS Inc.)
Unreliable ESP dumping can lead to shorting out of the transformer rectifiers and the associated missions troubled by losing a cell of your ESP. Also, inconsistent dumping can put operators directly in harm’s way just since they are not consistent in the way they dump; and then indirectly, if the dumping leads to unit shutdown which will expose them during the shutdown. So, reliable operation of those hoppers is important.
At CHS, we have two dry ESPs on both of our operating units. We have not really noticed a difference. That catalyst morphology has much to do with whether they dump or not; but it is an unrefined operation, so I cannot really speak about it. What I can tell you is that as you let the catalyst accumulate in that hopper, it forms an insulating barrier. The thicker that insulating barrier gets, the more chance you will have for a temperature gradient across that barrier to be less than the bulk temperature of the ESP. So, as you let it build, the likelihood of condensation increases dramatically. Condensation is the leading cause of catalyst hopper issues.
Now I will talk about the importance of proper design and operation of ESP hoppers to ensure that they dump correctly. Insulation is important on your hoppers. Pay very close attention to manufacturer’s recommendations around the corners. The contractors will often get that wrong. Also, check that the heater grids are properly installed: the strike plates, level indicators (typically nuclear), and vibrators you use for evacuating the hopper. We also have fluffing nitrogen connections above the knife gate valves that may help get catalyst moving. We have never used them, but they are there.
Regarding the operation, do not let catalyst accumulate in the hopper. Empty each hopper early and often; and when there is an upset, empty the hopper more often. You cannot do it enough. Pay attention to the sensory indicators. A good operator can tell if the hopper is empty or not if it is not rattling right. Utilize strike plates to hear the difference between the sound of an empty drum and a full one. Next, monitor those hopper temperatures; and then, do grid checks. These hopper heater grids have multiple patches. You can lose one patch and have a cold spot, so make sure you are checking each one of those grids on a regular interval: maybe quarterly. I think we do ours every six months. We check those grids to ensure they are working right before the winter hits, because they can short out. We have lost patches and been able to catch it that way. The bulk temperature of ESP inlet at 425°F or greater. If you are too aggressive on your sootblowing activities for your waste heat boiler, sometimes you will get that temperature a little too cold. The ESP does not perform well when the flue gas is too cold.
DINKEL [Marathon Petroleum Corporation (MPC)]
I agree with Darin’s points about making sure your hopper heaters are working and not allowing the hoppers to back up. I will add a strategy we use internally. One of the newer units is doing biannual PM (particulate matter) audits with the manufacturer coming in to perform a complete review of the ESP, including looking at all the cells and basically going through and tuning the cells to optimize performance. On an older unit that we just retired last year, we got to the point where we were doing quarterly audits on it to make sure we could maintain our environmental compliance.
FEDERSPIEL (W.R. Grace & Co.)
We looked at what could be complicating fines handling out of an ESP. We might be able to break that down into some mechanical integrity issues where it is possible that internal abrasion is impacting your ability to offload due to long-term operation. If the valve fittings were misaligned due to thermal cycling, or if catalyst particles fouled the seats of the valve guides, then that might also impact your ability to withdraw the catalyst. I think this is the first time the panelists are going to disagree. I get to say that catalyst PSD (particle size distribution) and morphology, I believe, do play a role in the ability to move those fines material out just by the fact that an irregularly shaped particle has a higher surface area. And because these are fines, you know the surface area-to-mass ratio is a little higher and gets a little more cohesive as we increase that surface area to mass ratio.
The last part of the question is about safe handling. Using proper PPE (personal protective equipment) is going to include goggles and a face mask. Also, make sure you are properly grounded before any operators to do anything with the ESP.
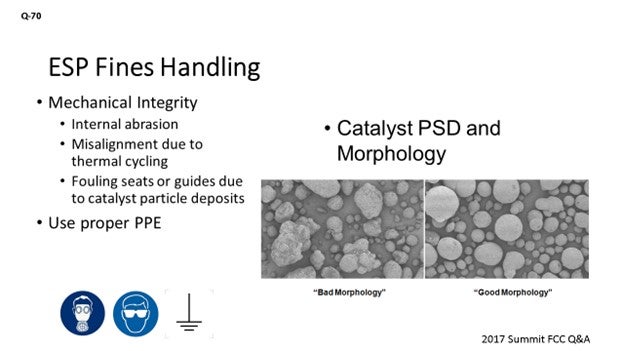
MICHAEL FEDERSPIEL (W.R. Grace & Co.)
Electrostatic precipitators (ESPs) represent an effective medium for particulate emissions control and are, therefore, commonly used within the FCC industry, especially in North America. Although ESPs are not designed to capture all of the catalyst particles present in the regenerator flue gas, they usually exhibit sufficient performance to successfully reduce the particulate content in the flue gas below 50 mg/Nm3. As the question suggests, fluidization and mechanical integrity issues can significantly hinder the withdrawal and handling activities of catalyst fines.
With respect to mechanical integrity, the most common issues correspond to malfunctioning catalyst discharge valves to the collection hoppers due to any of the following causes, among others:
- Internal abrasion throughout long-term operation,
- Misalignment of the valve fittings due to thermal cycling, and/or
- Fouling seats or guides due to catalyst particle deposits.
The electrode and collection plate rappers can also experience mechanical integrity issues that can significantly hinder catalyst withdrawal efficiency. These mechanical rappers help maximize the recovery of the ESP fines while properly preserving electrode efficiency and performance throughout long-term operation. Complete failure, or suppressed performance of these rappers, generally reduces the number of fines withdrawn from the system at constant particulate loadings and can significantly shift the particle size distribution (PSD) of the withdrawn fines towards a coarser profile. The reduced capability of withdrawing fines is usually accompanied by gradual increases in particulate emissions at the stack beyond the normal or allowable ranges. The mechanical integrity of the rappers can be affected by thermal cycling over time, as well as fouling issues stemming from the ingress of fines.
Operating conditions also influence the mechanical integrity of the ESP. Sudden thermal cycles, such as those associated with an emergency trip of the unit or sudden bypass of the ESP train, can increase the threat of mechanical integrity deficiencies associated with buckling or thermal expansion. Electrical or pneumatic supply deficiencies have also been reported, although they can be mitigated through redundant supply systems and/or onsite spare parts for the critical components. These types of mechanical failures have generally exhibited frequencies of zero to five times per planned turnaround cycle. Other mechanical or electrical supply issues are also commonly reported, but these tend to impact ESP performance to a much greater extent than the capability of handling fines.
The morphology and PSD profiles of the ESP fines will have a strong impact on the fine's withdrawal efficiency. Cyclone performance within the regenerator plays a major role in ESP performance and the corresponding fluidization properties of the ESP fines. Healthy cyclone operation typically results in average particle sizes (APS) in the range of 15 to 30 microns, depending on the regenerator design and overall hydraulic profile. Adequate cyclone performance helps maintain a manageable particulate loading to the ESP while sustaining a healthy PSD profile for the ESP fines. Excessive loadings to the ESP, over an extended duration, can have the following impacts:
- Increased erosion of the internal ESP components and fines withdrawal fittings.
- Increased quantity and APS (in the absence of catalyst attrition) of the ESP fines, which can improve fluidization of the withdrawn fines, but hinder overall capability due to the higher amount of material; and/or,
- Inadequate cooling of the ESP fines before the collection bins become full, which can constrict logistics during operation.
Excessive attrition within the reactor-regenerator system or the regenerator flue gas train can significantly reduce the APS of the fines, essentially increasing the concentration of microfines and fractured particles. These microfines and fractured particles tend to agglomerate, preventing smooth flow of the ESP fines into the collection hoppers. This type of fluidization issue is more prevalent once the APS of the fines drops below 15 microns. The jagged edges caused by catalyst fracturing can be identified by SEM analyses of the ESP fines samples. Even with a healthy PSD profile in the fines, agglomeration can occur due to other flue gas system failures, such as a flue gas cooler leak. The steam and boiler feed water in contact with catalyst fines can quickly lead to undesired catalyst agglomeration. Further, in extreme cases, acid dew point corrosion may be observed. These types of flue gas cooler leaks can be detected through sudden increases in the process-side pressure and much higher metals deposits on the ESP fines than those of the circulating e-cat inventory. The affected flue gas cooler tubes, or banks, should be isolated as quickly as possible to mitigate further erosion and downstream issues.
With respect to safe handling of the ESP fines, the industry Best Practices involve adequate use of PPE and easily accessible manifolds for the fines withdrawal system. In addition to the standard PPE requirements for refinery operations, fines handling activities should be accompanied by safety goggles, a respirator mask, and adequate equipment grounding facilities.
BRYAN DINKEL [Marathon Petroleum Corporation (MPC)]
Within the MPC system, we have only one operating unit with an ESP and we utilize gravity dumping into roll-off bins. This ESP is a relatively new piece of equipment that followed recommended design guidelines from the manufacturer. We do not have handling issues, as long as hopper heaters and vibrators are maintained. As a precaution, we have the equipment manufacturer conduct biannual field assessments to perform preventative maintenance (PM) and assurance that the equipment is functioning properly. During the design phase of a TSS (third-stage separator) project, MPC funded a hopper study that was completed by Jenike & Johanson. The goal was to optimize hopper selection and design angles based on their own analysis to determine physical properties of the catalyst fines. This strategy could be applied to ESP hoppers as well.
We recently retired a unit that had an old ESP which had design, maintenance, and operational deficiencies. That unit battled frequent issues with elevated opacity due to hoppers backing up. A hopper backing up poses multiple risks to the ESP performance, including being the cause of breaking wires, shorting out cells, and re-entraining fines into the flue gas flow path. A focused response was implemented to address these failures. We utilized quarterly preventative maintenance audits to resolve most of the issues. These audits included onsite electrical engineers, I&E (instrumentation and electrical) technicians, Operations and Maintenance personnel, and the equipment supplier. Key findings included the following:
- Hopper Heaters: The majority were not functioning, which allowed moisture to condense in the hoppers. The mix of catalyst fines and moisture resulted in plugging in the hoppers, an inability to properly empty the catalyst to the roll-off bins, and backed-up catalyst into the ESP. These were repaired to keep the temperatures hot.
- Hopper Levels: The manufacturer recommended installing hopper level indicators to keep from running catalyst levels too high. The short-term solution implemented was to increase the frequency of operator rounds to dump hoppers into the roll-off bins.
- TR (Transformer/Rectifier) Tuning: Quarterly re-tuning of voltage to TRs was completed to optimize collection efficiency.
- Roll-Off Bins: Attention to detail was elevated regarding connections from the hopper into the roll-off bins and the integrity of bag filters on the roll-off bins to keep the catalyst fines in the enclosed system.
- Cell Design Dimensions: With rate increases over the years, the equipment no longer met the recommended design aspect ratio from the manufacturer, resulting in less than the recommended residence time. A recommendation was made to replace the equipment to address this problem. Talk to your manufacturer about his/her recommended dimensions relative to your operating conditions.
DARIN FOOTE (CHS Inc.)
At CHS, we have dry ESPs on both of our operating units. Both utilize gravity dumping into contained roll-off bins.
Our experience is that catalyst fines content or morphology does not have a noticeable effect on whether or not ESP fines will dump from the hoppers. Catalyst that is allowed to accumulate in the hoppers can act as an insulator to the bulk temperature inside the ESP. As the accumulated catalyst piles up, the likelihood of condensation increases near the wall. Condensation is the most common cause of catalyst dumping problems. The following are important elements of design and operation that help ensure safe and reliable hopper evacuations.
Design
- Insulation: Proper insulation design is essential. One common pitfall is for insulation contractors to ignore the manufacturer’s insulation standard around corners, creating cold corners.
- Heater Grids: Ensure that properly sized hopper heater grids are installed correctly.
- Strike Plates: Confirm that strike plates are accessible and used on a regular basis.
- Vibrators: Check that they are installed to the manufacturer’s standard.
- Level Indicators (typically nuclear): Confirm that they are installed properly and accessible for maintenance.
- Fluffing Nitrogen Connection above the Knife Gate Valve: Use this option as a last resort to resolve dumping issues. We have these connections but have never used them.
Operation
- Do not let catalyst accumulate. Regular hopper evacuation is essential during normal operation. Frequencies should be increased around startup, shutdown, and malfunction events.
- Pay attention to sensory indicators when dumping. Seasoned operators can tell an empty hopper by the sound of the hopper vibrator. If you suspect an incomplete evacuation, use the strike plates and/or fluffing nitrogen connections.
- Monitor hopper temperatures regularly.
- Do regular electrical checks of the hopper heater grid to ensure that all circuits are working.
- Verify that ESP bulk inlet temperature is at least 425°F. If your sootblowing program on the regenerator waste heat boiler is too aggressive, this practice can decrease the bulk inlet temperature and increase the likelihood of condensation.
Question 81: Under what conditions is iron on FCC catalyst mobile, and how does this affect catalyst performance?
DE GRAAF (Johnson Matthey Process Technologies)
I think that the last statement from the floor was an excellent introduction to this question about iron mobility. Surprisingly, I was the only one on the panel who was willing to take this question; but I do expect some reaction from the floor hereafter. The first reports from iron poisoning come mainly from the 1990s. Iron was used in drilling liquids for oil recovery. What happened in a couple of units is that when the iron on the catalyst increased, you would see a decrease in ABD and an increase in slurry yield because the outer surface was completely closed off. You can see that because of the drop in ABD, there was some erratic catalyst circulation. And frequently, you can see an increase in SOx emissions because iron on the catalyst acts as a sort of inverse SOx additive. It reacts with H2S in the riser, drags it to the regenerator, and oxidizes down to SOx.
There are a lot of papers out there and many beautiful pictures, like the sort of Death Star shown in the bottom left-hand picture. You can see that the catalyst is covered with nodules. The nodules typically contain an iron top. The glassified outer surface of the catalyst is about 0.50 microns and can be up to 3 microns. When you look into that outer layer, you see a lot of very small magnetite iron oxide crystals. The problem is not so much iron on catalyst as it is the amount of added iron from the feed. When you study iron-poisoned catalysts with transmission electron microscopy, you see the iron present in small crystals. The crystals form agglomerates of about 10 to 30 nanometers, and they tend to cluster at the outer surface of the catalyst. That outer surface of the catalyst is eutectic.
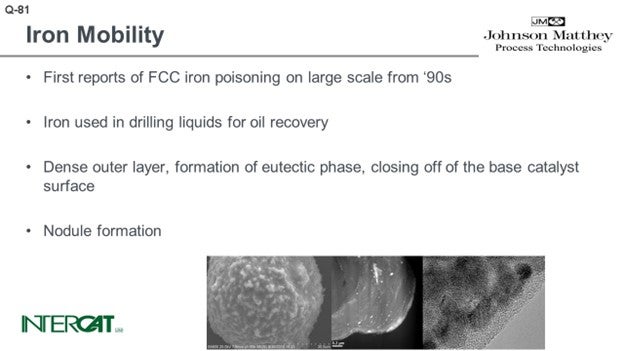
The problem with iron – even at level of, say, 0.3 wt% added iron – is that all of the added iron is present at the outer surface, which means that the concentration of iron at the outer surface can be quite high: easily be 4 or 5 wt%. It is concentrated and causes a eutectic to form. When you see that nickel or copper touch upon the catalyst or deposit on the catalyst, there can be interaction with alumina. The nickel or copper form nickel aluminate or copper aluminate and have a very good chemical bond at the base catalyst; vanadium, less so. Iron oxide also has no affinity with any component on the base catalyst. So, what you see is a sort of soup of silica with iron oxides floating in it.
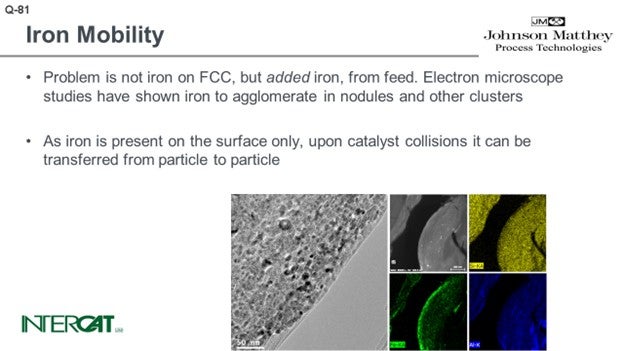
So on one hand, what determines metal mobility and what is the affinity of the metal to bind with the base catalyst? On the other hand, what is the possibility for transfer? If you have a sticky outer surface in the FCC, you will have multiple particle-to-particle collisions. By particle-to-particle collisions, as we have a sticky surface, you will observe the transfer of some of the crystallites of those iron crystallites. We used scanned electron microscopy to look into hundreds of particles, and we did population studies. If a metal has infinite diffusivity, it can diffuse up to the speed of light from particle to particle in an FCC unit. What you would expect is that every particle would have the same concentration. Vanadium has a low affinity for binding to an FCC catalyst particle. What you see at the bottom left-hand side graph is a Gaussian distribution of vanadium and distribution of the particles.
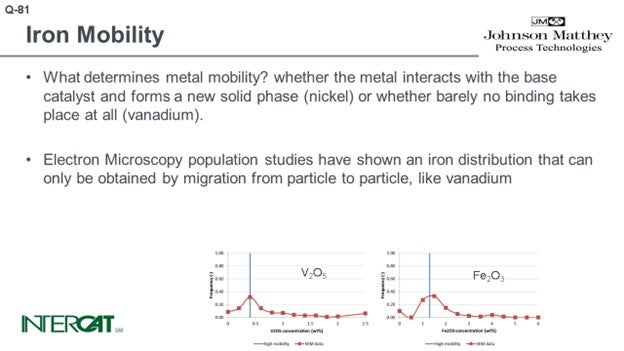
At first, we were also very surprised to see that in every case of iron poisoning we observed, the iron had a similar Gaussian distribution curve. In hindsight, we were not so surprised looking into the mechanism of how iron migrates and the affinity of iron to bind one particle because the affinity is just not there. We did some laboratory experiments and investigated whether we could mimic what happens in a unit suffering from iron poisoning. Under mild steaming conditions, we saw that there is migration happening when you steam the iron-poisoned catalyst. If you take 75% of this iron-poisoned catalyst and 25% inert, by using a moderately mild steaming protocol, we can show migration of iron to the inert particles. Under severe steam protocol, we got the result you see on the slide. We saw a transfer of iron and formations of nodules on inert that initially did not have any iron on it.
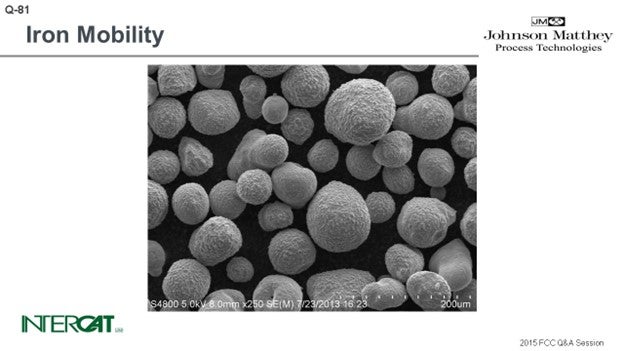
KENNETH BRUNO (Albemarle Corporation)
Indeed, this is a very challenging and complex story and situation, and in fact, contentious. As Adam said earlier in response to the previous question, the source of iron is critical: whether it is inorganic or organic. Organic iron will be the more harmful of the two.
Regarding mobility, our results are different than what Bart (Johnson-Matthey) described. We have seen some evidence of mobility, but we feel it is largely mechanical mobility; analogous to dust particles interacting, for example. However, we have found no conclusive evidence of what we call atomic mobility. Even maybe more importantly, we have studied many cases and have no conclusive evidence of iron preferentially adhering to a specific catalyst or additive particle.
Question 80 actually referred to accessibility or diffusion being important. And of course, we agree. There was also a comment about the importance of pore architecture. Of course, pore architecture is important. But when you are dealing with iron, the surface accessibility or surface architecture is even more critical.
So, with those points, again, the effect of Fe is a very challenging and complex problem. There are many different and competing thoughts on this topic. We have more data and details provided in the Answer Book. So again, we encourage you to consult the Answer Book. Thank you.
DE GRAAF (Johnson Matthey Process Technologies)
In response to Ken’s comment, the inert we used in this question is a silica/alumina-based inert. It was clay sprayed. It did not contain a metal trap. So, what we observed here was a transfer of nodules of iron from an iron-poisoned catalyst to a completely inert particle and formation of nodules on the clay particle.
MELISSA CLOUGH (BASF Corporation)
You might have seen the article that was published today in the AFPM Daily. I will just talk about a couple of points. BASF has also looked at iron mobility and the effects of iron. We are working with a number of refiners here in the States, and we are also looking at units in Asia and Europe. We evaluated it in a number of ways. First, we used scanning electron microscopy. We have also done separations and looked at the iron in the different fractions. Another technique is literally using a magnet and pickup catalyst with high iron to leave the low iron behind.
We then compared the iron distribution in the different fractions as shown by the three methods. In most cases, we see that iron mobility is very different than that of nickel and vanadium, and we definitely do not see very high mobility. I included some numbers in the article, but just in qualitative words. The mobility we have seen is more similar to nickel than to vanadium.
DE GRAAF (Johnson Matthey Process Technologies)
We have done similar experiments, including sink/float experiments. We typically see that in old layers, there is a similar amount of iron present. When you study these catalyst fractions with electron microscopy, you see every layer has a Gaussian distribution present again. So even though the amount of iron per layer is fairly constant for every fraction of the sink/float, we have observed a Gaussian distribution of metals.
RAMA RAO MARRI (CB&I Lummus Technology)
So far, I think we have discussed mostly the catalyst solutions. Thank you all for contributing information about the catalyst, but another aspect is the hardware. The ultimate effect of the iron, mainly when it is a change of the feed out of crude slate to the refinery, is the increase in the bed temperature. That is the fundamental change. So, when it changes, the dense-bed temperature catalyst reduces, and conversion is affected.
An option we adopted in one of the refineries that experienced this situation was to implement a long-term solution to further our technology from changing out the disc condoner. This is typical technology that helped them to ease out the increase in resid bed temperature. It also helped increase the catalyst issued and carried out the conversion loss. So, this is one of the catalyst solutions that can be considered.
BART DE GRAAF (Johnson Matthey Process Technologies)
The first reports of FCC iron poisoning on a large-scale date from the 1990s. Iron was used in drilling liquids for oil recovery. Iron poisoning results in a loss of activity and an increase in slurry yield. The apparent bulk density of the catalyst decreases, which causes a drop in pressure differential over the standpipes and can lead to erratic catalyst circulation.
The effect of iron poisoning has been highlighted in many papers including electron micrographs of FCC particles covered with nodules. The pictures show further a dense outer layer due to the formation of a eutectic phase. This dense outer layer closes off the base catalyst surface.
The iron in the FCC catalyst itself is not the problem, but added iron is iron from feed. Even at levels of 0.3 wt% added iron, some catalysts are already poisoned by iron. The 0.3 wt% is very unevenly distributed over the catalyst; all the added iron is at the catalyst surface where it easily reaches 4 wt% and is enough to create the eutectic dense surface layer.
Iron from feed is deposited as single atoms on the base catalyst. Electron microscope studies have shown iron to agglomerate in nodules (caps of nodules typically consist of iron oxide) and other iron oxide clusters. These iron oxide clusters show that iron atoms are mobile on the catalyst surface. Added iron oxide is only present in the dense outer layer of the particle; it does not diffuse deeper into the FCC catalyst particle. The outer surface is somewhat sticky because of the eutectic. As iron is present on the surface only, upon catalyst collisions, it can be transferred from particle to particle.
What determines metal mobility? Metal mobility is determined by the possibility of the metal interacting with the base catalyst and whether it can form a new solid phase (nickel) or whether barely any binding takes place at all (vanadium). Iron oxide does not bind to any base catalyst component.
Electron microscopy population studies have shown an iron distribution that can only be obtained by migration from particle to particle, like vanadium. These results have been obtained by studying several hundreds of e-cat particles and comparing their average metal concentration on particles. As iron distribution due to its agglomeration is very uneven over catalyst particles, it is essential to study average concentrations per particle and compare average concentration per particle versus the number of particles with this concentration. As comparison, in order to study whether the flue is contagious, it will be more relevant to establish the core temperature of 5,000 people than to study one person and measure this person’s temperature at 5,000 different spots.
KENNETH BRYDEN and SHANKHAMALA KUNDU (Grace Catalysts Technologies)
Iron (Fe) is present in FCC catalyst as an element in the clay used in manufacture. Hence, the iron content of the fresh catalyst is dependent on the clay source and clay content of the catalyst and will vary from supplier to supplier and catalyst to catalyst. Additional iron comes into the FCC unit from contaminants in the feed and this additional iron can have adverse effects on catalyst performance when it results in an iron-rich glassy shell on the surface of catalyst. The shell inhibits diffusion thereby lowering conversion and increasing slurry yield18. One of the first descriptive scientific publications on the subject of iron mobility was the Grace paper “Mechanism of Cracking Catalysts Deactivation by Fe,” that was published in 2004 in Volume 149 of Studies in Surface Science and Catalysis19. In this paper, sink/float density separation was used to separate equilibrium catalyst into different age fractions. For a unit with a low to moderate amount of deposited iron, the iron showed a sharp, non-uniform distribution with catalyst age, suggesting that the iron had much lower mobility than V and Na in the unit and that iron mobility was similar to Ni (Figure 1).
Figure 1. Distribution of Fe, Na, Ni and V on e-cat fractions from a unit with low to moderate amounts of Fe separated by a sink/float method. Fe on e-cat was 0.74 wt% Fe2O3. In this case, Fe and Ni content of e-cat increases sharply with age indicating that the two contaminants are not mobile. Na and V content of e-cat does not change as much with age, suggesting increased mobility.
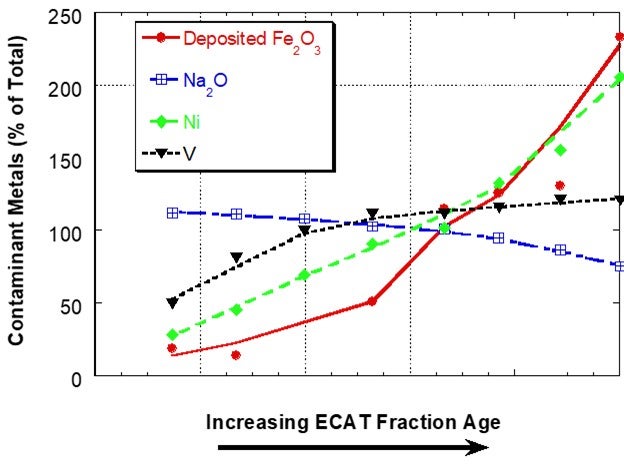
However, for a unit with a very high level of deposited iron, the iron distribution was relatively uniform between the different age fractions, suggesting that the interparticle mobility of iron was the same or higher than the vanadium mobility (Figure 2). In sink/float analysis of e-cat from additional units, Grace has found that iron can be mobile in some units and for those units the iron mobility is typically of the same magnitude as vanadium mobility.
Figure 2. Distribution of Fe, Ca, Na, Ni and V on e-cat fractions from a unit with high amounts of deposited Fe separated by a sink/float method. Fe and Ca levels on e-cat were 1.91wt% Fe2O3 and 0.15wt% CaO (calcium oxide). In this case, the iron is relatively uniformly distributed among particles of different ages, suggesting mobility.
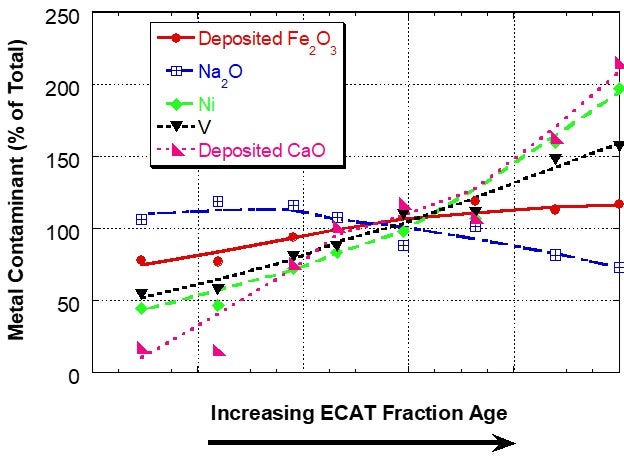
In laboratory experiments where iron rich e-cat is steamed with fresh catalyst without depositing iron, we have found that some iron migration can occur to the fresh catalyst. The level of iron transfer increased with increasing steaming temperature and increasing contaminant iron level. In these experiments, we did not observe any reduction in the iron nodules present on the iron-enriched e-cat, and no nodule formation was observed on the fresh catalyst.
What is not fully understood is how iron moves from particle to particle within a unit and if this movement has any effect on catalyst performance. Detailed SEM and EPMA (electron probe microanalysis) analysis of e-cat particles has found that deposited iron is always on the exterior surface of the particle and is not present in the interior of the catalyst particle. This suggests that iron does not migrate within an individual particle. If the iron transfer was via a gas-phase diffusion process, it would be expected to also move within an individual particle, which is not observed. The most likely means of iron mobility is physical transfer upon particle/particle contact. In the 2004 “Studies in Surface Science and Catalysis” paper, Grace noted that deposited iron in a unit comes from different sources (organic iron, finely dispersed sub-micron colloidal iron, and iron particulates) and that iron migration may be due to loosely attached iron rich dust migrating from particle to particle. It may be that the iron species which can move from particle to particle in a unit are not the same iron species which are responsible for the nodule formation on the surface of e-cat. The mechanism of iron migration in a unit is an area of ongoing study. Iron migration appears to be dependent on unit conditions and the types of iron present in the feed.
To manage iron poisoning, refiners should reformulate to more iron-resistant catalysts and consider higher fresh catalyst additions. Catalyst design can be optimized to resist the effect of contaminant iron and calcium. High alumina catalyst, especially catalyst with alumina-based binders and matrices, such as Grace’s MIDAS® and ACHIEVE® catalyst families, are best suited to process iron- and calcium-containing feeds because they are more resistant to the formation of low-melting-point phases that permanently destroy the surface pore structure. Optimum distribution of mesoporosity (pores in the 100 to 600 Å size range) also plays a role in maintaining performance because diffusion to active sites remains unhindered, even with high levels of contaminant metals. The resistance of MIDAS® and ACHIEVE® FCC catalysts to iron and calcium poisoning has been demonstrated in numerous commercial applications20,21,22.
Some refiners also consider the use of flushing e-cat to temper Fe poisoning. This strategy can help limit the effect of Fe on fresh catalyst but comes with several caveats. One must make sure the e-cat in use is not contaminated with Fe (Fe poisoning can occur at levels as low as 0.2 wt% added Fe); additionally, the quality of the e-cat may require a change to the fresh catalyst strategy (either formulation or addition rates) to maintain desired unit performance.
In summary, iron can be mobile in an FCC unit, but it is unclear how iron mobility affects catalyst performance. Not all of the factors influencing iron mobility are understood, but it appears to depend on iron concentration, temperature, and the types of iron contaminants present in the FCC feed.
MELISSA CLOUGH (BASF Corporation)
When looking at iron mobility, or any metal mobility for that matter, it is important to differentiate between intraparticle mobility (within a single catalyst particle) and interparticle mobility (from particle to particle). It is also important to understand that the answer is usually not a clear “yes” or “no”; as with all FCC questions, it depends. So, trying to quantify the answer is more important than a “yes” or “no”. Looking at intraparticle mobility, we can quantify this type of mobility by using scanning electron microscopy (SEM) data of cross-sectional areas of catalyst particles. Comparing the amount of contaminant metal on the outside versus on the inside gives us a Peripheral Deposition Index (PDI).23 For context, vanadium typically has PDI values of approximately 1 indicating close to uniform content of vanadium throughout the particle and thus high intraparticle mobility. We have seen iron PDI values ranging from 4 to over 7, indicating low intraparticle mobility and similar to the deposition profile of nickel. For interparticle mobility, we can use a variety of techniques to study bulk e-cat. Metals analysis of sink-float separated catalyst particles (where fractions are separated based on age) shows a standard deviation among fractions for iron being between those of vanadium (high mobility) and nickel (low mobility). Looking at bulk e-cat in SEM, you can clearly see a distinction between new (no iron) and old (iron) catalyst particles via nodulation and surface mapping, indicating that the mobility profile of iron is distinctly different than that of vanadium. In summary, across a number of commercial units studied, iron does not show high mobility like vanadium. Its mobility is low and closer to that of nickel.
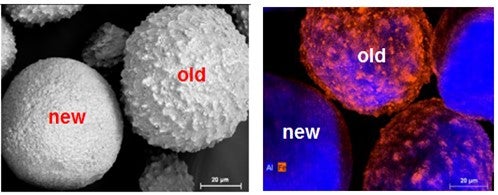
Left: SEM morphology of a new and old catalyst particle, differentiated by iron nodulation
Right: SEM surface mapping (alumina in blue; iron in red) of old and new catalyst particles
Units with iron-poisoned catalysts can face a number of concerns either from the chemical or physical effects of added iron. The chemical effects are often minor and include higher hydrogen and coke due to the dehydrogenation activity of iron, mild CO promotion, and the transfer of sulfur from the reactor to the regenerator, which can increase SOx emissions. The physical effects include nodule formation, which can impact catalyst circulation and vitrification, which can reduce surface area. Severe poising leading to surface blockages is the most common concern. Surface blockage can cause a drop in conversion with increasing added iron, leading to higher slurry yields. The amount of iron that causes significant surface blockage to result in a loss of conversion is another “It depends” answer and depends heavily on the type of catalyst. Catalysts with optimized porosity, especially surface porosity, give improved iron tolerance. BASF practices in-situ manufacturing (as opposed to incorporated manufacturing) which has been shown to have improved iron tolerance (BASF survey completed in 2001). Based on surface morphology, in-situ manufactured catalysts have high surface porosity which can withstand a higher degree of fouling due to iron. BASF catalysts have been successfully used commercially with above 2 wt% e-cat total iron, or 1.5% added iron.
GEORGE YALURIS, KENNETH BRUNO, and RYAN NICKELL (Albemarle Corporation)
Iron is a contaminant metal often found in the FCC feed and can be either organic or inorganic in origin. As the feed evaporates and cracks, iron deposits on the catalyst. Only organic iron – either molecular or colloidal – significantly reacts with catalyst components such as silica and other feed contaminants such as calcium and sodium, forming eutectic phases which sinter and destroy the surface porosity of the catalyst. As the surface pores begin to close, the large feed molecules encounter increased diffusional resistance to access the catalyst’s interior. The catalyst particles get encapsulated by the formation of a vitrified, low-porosity, iron-rich ring (an egg-shell formation) and lose their ability to absorb, evaporate, process, and crack feed molecules. The result is reduced bottoms upgrading and conversion.
As the surface structure is destroyed, the catalyst becomes nodulated (which causes a decrease in the ABD). The iron ring typically penetrates no more than 5 m deep into the particle. If iron were atomically mobile, it could easily penetrate inside the catalyst particles and get dispersed to much lower concentrations, and we would not observe an iron ring around the catalyst particles. It is therefore clear that no molecular or surface diffusion mechanism exists that enables iron mobility.
It is important to realize that iron in the FCC feed can also manifest as inorganic particulates ranging from dispersed micron sized to larger, even catalyst-size particulates. The source and composition of these particulates can vary from highly enriched iron formations (perhaps the result of corrosion and erosion of hardware) to high iron content soil/clay particulates with intricate yet decisively non-FCC catalyst shapes. We show an example of such iron-rich particulate contaminant in the below SEM/EDX chemical mapping image.
SEM image of a high-iron clay particulate found in an FCC unit
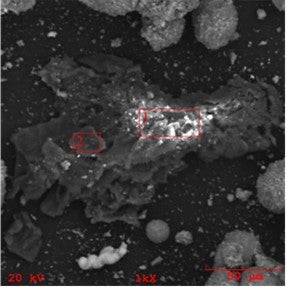
If small enough, these particulates can attach themselves to the catalyst surface. We show below an example of a larger high-iron particle attached to a catalyst particle, noting that most of the feed particulate contaminants that can attach to the catalyst particles are smaller than a few microns. This kind of particulate iron contamination may look bad in SEM images, but it actually does only minor damage, if any, to the catalyst as large portions of the exterior surface remain unaffected.
Iron particulates can break away and move from one catalyst particle to another, the same way small dust particles can move from one larger particle to another. One can also expect that as catalyst particles abrade or break into smaller ones, sections of the exterior surface rich in iron can form smaller micron-size particulates which also can move from one catalyst particle to another. In other words, iron can demonstrate “mechanical mobility”. Since these fine particles (regardless if they come with the feed or are generated by attrition mechanisms) are easily entrained, the fines of units with iron contamination are often enriched in iron; meaning, they contain more iron than the e-cat which generates them.
Below is an SEM/EDX chemical mapping of an FCC catalyst particle cross-section which shows a large high-Fe particulate attached to it, as well as several smaller (1 to 2 m) particulates. Areas of high iron are indicated by green, yellow, and red colors.
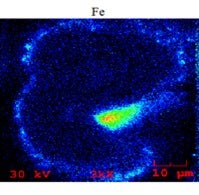
The mechanical transfer of iron particulates from one particle to another can appear falsely significant, since relatively fresh catalyst particles can have some iron on their exterior surface and even nodule formations like what we typically see in older iron-deactivated catalyst particles. However, as it should be apparent now, the formation of these nodules on the exterior surface of the catalyst particles is a necessary but not sufficient condition that could result in bottoms cracking and unit conversion loss. Nodules can form by organic (molecular and colloidal) and inorganic (finely dispersed and particulate) iron; yet the impact on the catalyst and unit performance can be dramatically different depending on the nature of the iron contamination. Indeed, we have seen resid cracking units where as little as 2000 ppm added iron can cause significant catalyst deactivation; while in others having added iron of 5000 ppm, there was no effect on bottoms cracking or conversion. When we investigated these units, one of the key factors we found determining the effect of iron on catalyst and unit performance was proven to be the nature of the iron in the feed.
The mechanism we described here for iron particulates moving from one particle to another is a non-specific and random mechanical process. Exhaustive investigations to date, conducted by Albemarle, have demonstrated that there is no catalyst or additive currently being used in FCC units which preferentially pick up iron. The most effective way of resisting the effects of iron contamination is by leveraging highly accessible catalysts with an alumina matrix (such as Albemarle’s ADM-20 bottoms cracking alumina). These catalysts provide the open-pore architecture with larger pore mouths on the particle surface which are harder to bridge and block. They also resist the formation of iron-silicon- calcium-sodium eutectic phases which sinter and destroy the surface pore architecture of the cracking catalyst particles.
Question 21: We are observing fouling of our feed/effluent exchangers that has impacted heat transfer and restricted feed. What are potential contributing causes and how can we mitigate?
ROBERT STEINBERG (Motiva Enterprises)
There are many things that can contribute to fouling of feed/effluent exchangers. Fouling can occur on either the feed or product side of the exchangers.
Possibilities sources of fouling on the feed side include:
• Dissolved O2. Oxygen can get into feeds if they come from a tank that is not N2 blanketed, this is especially likely if feeds have been imported from another site via a barge. Oxygen can also be present if a feed come from a vacuum tower with an air leak. Corrosion inhibitors or oxygen scavengers injected into the feed as far upstream as possible may help. The best method to remove oxygen is to add an O2 Stripper on the stream that contains oxyg
en.
• Caustic. Small amounts of caustic that was not water washed can lead to severe fouling.
• Particulates, scale, corrosion particles. FeS scale is often found in refinery streams. If the source is known, corrosion inhibitors may be able to reduce the amount of scale. Good feed filters may be able to remove some of the scale but FeS particulates can be small enough to pass through most feed filters.
• Dirty feed. Cracked feeds, especially coker gasoils, tend to be dirty and have small coke particles. Good filtering is essential. If not done at the upstream unit it needs to be done on the hydroprocessing unit. It is often a good practice to filter both places in case one of the filters is bypassed.
• Salt in Feeds. If crude oil is not properly desalted there can be salts left in heavy feeds. Salts from other sources can also be present at times. A water wash or a desalter can remove salts.
• High temperatures. High skin temperatures tend to increase fouling. High temperatures may be unavoidable when exchanged against reactor effluent, especially in the hotter shells. An exchanger design that increases velocity and promotes turbulence on the feed side will increase the heat transfer coefficient and reduce skin temperatures. Injecting hydrogen upstream of the exchanger will help.
• Low velocities. Lower velocities in the exchanger reduce pressure drop but lead to higher skin temperatures, make it easier for particulates to stick to tube surfaces and increase fouling. Injecting hydrogen upstream of the exchanger will help. Recycling hydrotreated product when the unit is turned down will maintain higher velocities in the exchangers.
• Cracked feeds. Cracked feeds have olefins and sometimes di-olefins which can polymerize and are more prone to fouling. Cracked feeds can be a particularly severe problem if dissolved oxygen is present. A selective hydrogenation unit or reactor can be used to saturate di-olefins at a relatively low temperature upstream of the main reactor before the feed gets hot enough for severe fouling to occur.
• Asphaltene precipitation. This is normally only an issue with resid units. Mixing different feeds, especially a lighter more paraffinic feed with resid, can create incompatible mixtures and cause asphaltene precipitation.
Reactor effluent is normally cleaner than reactor feed. Olefins get saturated and dissolved oxygen gets converted to water in the reactor. The reactor effluent will always have hydrogen which tends to keep velocities high. However, there are some possible sources of fouling on the reactor effluent side:
• Salt precipitation. H2S, NH3 and HCl are normally present. These form ammonium chloride (NH4Cl) and ammonium bisulfide (NH4HS) salts when temperatures fall below the salt formation point. The salt point is dependent on the operating pressure and concentration of H2S, NH3 and HCl. Salt point curves can be found in API Recommended Practice RP-932B Design, Materials, Fabrication, Operation, and Inspection Guidelines for Corrosion Control in Hydroprocessing Reactor Effluent Air Cooler (REAC) Systems. Typical precipitation temperatures are in the 300-400°F range for NH4Cl and around 100°F for NH4HS. In addition to fouling, these salts can be extremely corrosive if water is present. Dry salts are not corrosive but an intermittent water wash may be needed to remove them once fouling occurs.
• Polynuclear aromatics. This is normally only an issue with hydrocrackers, especially the 2nd stage of a two-stage recycle unit. If conversion is too high the PNA concentration can get high enough that they become insoluble in the oil. The lighter cracked products can cause PNA’s to precipitate in exchangers as the effluent cools and more of the naphtha range material condenses.
JOE RYDBERG (CITGO)
In our recent experience, fouling on the “feed side” of the feed/effluent exchangers in Naphtha units is due to corrosion products (Fe) entering with the feed, and processing recycled Naphtha’s particularly from LPG Caustic Disulfide separators. The recycled naphtha’s can have higher levels of Sodium and Salts (likely amine degradation products that build up in the caustic).
Other causes can be contamination of cracked stocks into the virgin stocks system. Exposure to oxygen will cause gum formation. Crude supply sources have unknown diluents. Refineries are now collecting more material from various refinery sources and rerunning as slops, for example introduction of flare gas recovery liquids, reprocessed as slop oil; re-processing/chemical cleaning liquids pumped to slop system.
Use of chemical additives (organic dispersant, antipolymerant, oxygen scavengers) can be used and are used within CITGO to mitigate fouling. Proper tracking of heat exchanger fouling is important and can aid in scheduling cleanings (requiring unit shutdowns) outside of turnarounds, during catalyst change-outs, etc. When dealing with especially challenging feeds and / or extending cycle length goals, installation of spare feed/effluent heat exchangers could be value added approach
Effluent side fouling typically is caused by inadequate water wash, presence of NH4Cl in addition to FeS. HCl can also react to create additional FeS in the presence of H2S.
ERIC LIN (Norton Engineering Consultants, Inc.)
In a hydrocracker with liquid recycle (could be single stage recycle or two-stage recycle), there exists the possibility of HPNA (Heavy Polynuclear Aromatics) buildup at the bottom of the fractionator. Although the overall conversion will decrease, the best solution is to have a dedicated bleed stream out of the unit (FCC is a typical destination) to prevent this buildup. High asphaltenes in the feed are usually a sure sign of HPNA production.
In a residue hydrocracker, the existence of sediment can cause similar fouling in these exchangers. Sediment can typically be mitigated with the use of slurry oil as a cutter (much easier to acquire for units that also have an FCC nearby).
SAM LORDO (Consultant)
Fouling in the circuit ahead of the furnace and furnace can be caused by inorganic solids, or polymerization of feed components (organic fouling). Mitigating fouling from inorganic solids, such as iron sulfide and other corrosion byproducts, sand and silt (from imported feedstocks) is primarily done using feed filters. The pore size is best at 1-5 micron. The filter can be cartridge style, sand filters. Some filter arrangement would have backwash capability.
Fouling downstream of the reactor may include ammonium chloride (NH4Cl). Typically, a well-designed Waterwash is used. The use of salt dispersants are also applicable where Waterwash is feasible
Organic fouling could be from:
• Stream that contain olefinic/diolefinic components which when exposed to elevated temperatures ass found in the hydroprocessing units
• O2 contamination of feed or feed component streams
Mitigation of this source of fouling can be done using an appropriate chemical additive, such as dispersant and/or antiploymerant.
MICHAEL PEDERSEN (Honeywell UOP)
Most hydroprocessing catalysts require a conditioning period at start of run to allow the active sites to stabilize. One aspect of this process is the common industry practice to avoid processing cracked feedstocks during the first few days of operation. Prior to conditioning, fresh catalysts have a high tendency to generate excessive coke when operated with reactive feedstocks or at normal unit operating severity. A short period of mild operating conditions can pay big dividends in overall catalyst cycle performance while high severity operation at start of run can have substantial negative impact on apparent catalyst activity and cycle length. In general, catalysts that are claimed not to require conditioning have been artificially inhibited prior to delivery.
Hydroprocessing catalysts encompass a wide variety of formulations, so a general set of conditioning guidelines is not applicable. For a specific catalyst system, instructions from the supplier should be followed.
SIMERJEET SINGH and RAJESH SIVADASAN (Honeywell UOP)
Fouling of feed/effluent exchangers in hydrotreating units is a common problem leading to throughput losses, increased energy consumption, unit downtime and maintenance expenses for exchanger cleaning. Fouling happens due to changes in feedstock quality, exchanger temperature, fluid velocity, degree of vaporization and exchanger configuration leading to formation of hard carbon deposits (coking), deposition of undesirable polymers (polymerization) and corrosion products.
For Coker Naphtha Hydrotreater:
• Feed quality issues:
Coker naphtha (CN), by the nature of thermal cracking reactions, contains free radicals, which react with diolefins and olefins to form oligomers and polymers. By itself CN presents a fouling problem in a NHT, however when combined with stored SRN there exists the potential for significant fouling. Storage of CN prior to processing can have disastrous results, as the combination of diolefins, free radicals, and oxygen (peroxides) can lead to rapid fouling on the feed side of the combined feed exchanger (CFE), the NHT charge heater, and the NHT reactor. This fouling can be serious enough to cause premature pressure drop increase along with loss of heat transfer due to fouling in a matter of days if not hours. The downtime associated with addressing this fouling costs the refiner time and money.
The highly reactive diolefins in CN are the four carbon and five carbon species, at the front end of the boiling range. Longer chain diolefins tend to be reactive, but less reactive than the short chain diolefins. Simply increasing the initial boiling point of CN (reducing the quantity of light diolefins) may reduce the tendency of CN to cause fouling. When cracked stocks with significant diolefin concentrations are present, it is UOP’s practice to include a diolefin saturation reactor as a first, low temperature reaction stage in a two-stage reactor system. In this reactor, most of the diolefins are saturated. This reactor is located in between CFE shells and its position is selected such that the inlet temperature is in the range of 320-370°F.
• Design considerations:
o Feed tank blanketing
o Design of feed tanks (Fixed/ floating roof)
o Hydrogen Injection to preheat exchangers
o “Over-Sized” exchangers for clean duty
o Exchanger velocities
o Dry Point location
For VGO HDT:
• Feed quality issues:
o Fouling is also experienced in units that run straight run feed only, so it is not just a phenomenon that requires cracked olefinic feeds.
o Fouling from asphaltene precipitation.
• Design considerations:
o Same design considerations as coker naphtha HDT except the dry point location.
o Thermal cracking of feed VGO in feed effluent exchanger can be of main issue if separate feed heating is being used as design feature over combined feed heating.
• Fouling Mitigation Strategies:
Many methods exist for managing fouling. The costs of these methods vary, as does their effectiveness. In order to choose the most effective method for managing fouling, an understanding of the source of foulant precursors should be established. Analytical methods are available that can be used to characterize a feed for gums, asphaltene or stability in the presence of oxygen. While these methods may or may not provide a complete solution to exactly where the fouling problem comes from, they may help to characterize the different feeds at a given site and help narrow down the probable root cause.
• Avoid oxygen contamination of feed.
Direct feeding – Supply feedstock to hydrotreater from upstream unit without using intermediate tankage.
Benefits:
o Eliminates residence time in intermediate tankage, thus minimizing formation of other free radicals.
o By far the cheapest solution and reduces working capital.
Risks:
o Lacks flexibility to accommodate swings in feedstock rate and unit outages.
Tank blanketing – If tanks must be used, they should be blanketed. Nitrogen is the best blanketing gas owing to its reliably low O2 content and ease of venting to atmosphere. Gas blanketed internal floating roof tanks are most effective in minimizing oil contact with O2 and evaporation losses to blanket gas.
Benefits:
o Commercially just as effective as direct feed and overcomes all the limitations.
o O2 cannot react if not in system, therefore should reduce foulant generation.
Risks:
o Cannot impact O2 brought in with import through other feeds
o Choice of correct seal for floating roof and its periodic checking and maintenance.
• Remove Oxygen from Contaminated Feeds.
Oxygen Stripper – Strips out free O2, including import O2 and removes the potential for further formation of peroxides. Common scheme is for ambient temperature hydrogen stripping of the feed to fuel gas system.
Benefits:
o Only feed streams exposed to O2 need to be stripped.
o Maximizing direct feed to the unit in combination with stripping the small O2 contaminant stream is generally more economical than stripping the complete feed stream.
Risks:
o Residence time, particularly in imports, may result in some polymer reaction occurring.
o Expensive option in terms of equipment, and is not so effective if the peroxides/ polymer has already been formed upstream of the stripper.
Injection of anti-oxidant chemical – Antioxidant chemicals have been used with a degree of success in some locations.
Benefits:
o Act as chain stoppers that react preferentially with O2 and peroxides, making them unavailable to take part in free radical polymerization reactions.
Risks:
o Although chemical treatment can help, it is not always successful and it tends to be most effective when the antioxidant is dosed into the upstream unit rundown ahead of the storage tank.
• Remove foulant/prevent laydown.
Hydrogen treat gas injection – Inject hydrogen treat gas upstream instead of downstream of preheat exchangers.
Benefits:
o Hydrogen gas increases turbulence and can also help to reduce polymer formation reactions.
o For VGO HDT hydrogen injection especially for units with separate heating of VGO will prevent thermal cracking of VGO.
o Avoid dry point in exchanger areas where the feedstock is completely evaporated towards dryness as severe fouling may happen. Polymer and gum tends to build up on the shell-side behind baffles, because of relatively stagnant zone. Evaporation of feed leaves less liquid solvent for the gums and gums get deposited. Most severe at the liquid dry point.
• Modify exchanger design – Modify exchanger internals, maintain high velocities in exchangers, appropriately oversize exchangers to lower high tube wall temperatures below the critical temperature required for coking or polymerization.
Parallel exchanger – Flexibility for bypassing and cleaning.
Benefits:
o Clean all exchangers on-the-run, extra exchangers mean no loss of throughput to clean.
Risks:
o No reduction in rate of fouling.
o Additional design features required (such as PRV’s) to safely by-pass/isolate exchangers.
• Anti-foulant chemical injection.
Benefits:
o A reduction in the rate of fouling.
Risks:
o Fouling mechanisms will still occur, probably downstream.
• Prevent corrosion
Corrosion resistant tube metallurgy – select appropriate tube metallurgy to prevent formation of corrosion products that aid the process of foulant formation such as naphthenic acids or high TAN feeds.
Benefit:
o Easy to implement for new unit and revamp of existing unit.
Risks:
o May not be the best solution as metallurgy upgrade is expensive and components other than tubes can still provide corrosion products to aid fouling.
IHSAN RAAD (Shell Catalysts & Technologies)
There are several types of fouling in Hydrotreating feed/effluent exchanger units, the three most common types in the industry are:
1. Inorganic particulates.
2. Organic deposits.
3. Ammonium salts.
Each type of fouling has its own characteristics and deposition locations. Knowledge of the type of fouling and the underlying deposition mechanism is essential to tackle the fouling problem. This can either be done by eliminating the root-cause, or by selecting a fit-for-purpose and cost-effective abatement approach.
1. Inorganic particulates: Inorganic fouling is mainly caused as a result of iron sulfide, sodium or coke fines that can either be carried from upstream units or generated in-situ in the preheat exchanger network. These foulants are:
• Iron Sulphide (FeS) and Iron Oxide (FeO, Fe2O3): Scales of iron oxide (FeO, Fe2O3) and iron sulphide (FeS) are generated as corrosion products within the unit itself but can also come from upstream units, intermediate storage and transport from well to refinery. Important corrosion sources are furnace tubes (hot sulphur corrosion), the CDU overhead condenser and the reactor effluent air cooler. Iron corrosion products in VGO’s are also associated with processing of naphthenic crudes.
• Sodium (Na): Na can come from brackish or salty cooling water (i.e. leaking heat exchangers) or from processing water-containing slops or imported feeds. Sodium in combination with iron has been known to promote coke formation under conditions of high temperature and low pp H2.
• Coke (C): Coke fines can be entrained from VBU’s and cokers, which cause mainly fouling of the feed side of the feed/effluent heat exchangers, furnace tubes and the top beds of reactors. VGO hydrotreaters might also experience coke formation due to a poor separation in the upstream HVU.
2. Organic deposits: The organic foulants are primarily gums formed as a result of processing cracked material and accelerated if the material is exposed to oxygen at any time. The types of foulants are:
• High di-olefin Content: Di-olefins (molecules containing (multiple) double bonds) are mainly found in product streams from (thermal) cracker units but can be present in other streams as well. At the right temperature level (~400-500°F) they polymerize readily to form gum-like substances often showing up as greyish flakes on the FEHXers. The rate of this reaction increases at higher temperatures, making the FEHX especially vulnerable. Elimination of feed streams with high di-olefin content is an easy way to reduce fouling but may not be preferred economically. If long periods of operation with high di-olefins content are expected, an option to avoid high fouling rates could be the installation of a low temperature di-olefin saturation reactor.
• Oxygen in Feed: Oxygen can form a range of different molecules when it is dissolved in a hydrocarbon stream (e.g. peroxides, carboxylic acids, aldehydes and other oxygenated compounds). Amongst other problems, these molecules can initiate polymerization reactions to form gums. Oxygen can enter a feed stream in several ways, including but not limited to air-breathing storage tanks, marine or surface transport vessels, leaks in equipment operating in sub-atmospheric pressure or faulty pump seals. One common point for oxygen to enter feedstock is during storage. Feed streams can be routed directly from unit to unit to avoid intermediate tank storage. Another option is to put in a bypass jump-over on the tank such that only the extra feed goes to tankage and the rest will bypass and go directly to the hydrotreater. All tanks used for storing hydrotreater feed should be nitrogen blanketed, also for straight-run feed. If this is not done, gum formation and other side reaction might happen in the tank itself. Other mitigation is to run it through a stripper, fractionator or distillation unit before introducing it to the unit in order to strip away both dissolved oxygen and the oxygenated compounds, like peroxides. A last option that is used to avoid oxygen related fouling is by injecting anti-oxidants into the feedstock. These compounds are only effective when they are injected prior to the feed stream coming into first contact with the oxygen. So, it should be ideally be injected at the source prior to transportation to site or send to storage. Also, good mixing of the anti-oxidant with the hydrocarbon streams is essential. Only then can they prevent gum formation during storage.
3. Ammonium salts: There are several types of salts that can formed in the effluent exchanger.
• If ammonia and HCl are present, ammonium chloride may deposit directly from the gas phase. The sublimation point in the process depends on the operating conditions (pressure and temperature profile) but also on the concentrations of ammonia (generated from hydrogenation of nitrogen compounds) and chlorides. Main locations of deposition are the feed/effluent heat exchangers (effluent side), the air cooler and recycle gas compressor valves. In the dry state this salt is not corrosive, but in areas where the water dew point is approached, the deposited NH4Cl salt will become moist and can be very corrosive (NH4Cl salts are hygroscopic, therefore the stream temperature must be maintained 15-20 C above the water dew point to assure dryness). Furthermore, apart from the danger of excessive corrosion, NH4Cl deposition can drastically increase the pressure drop and to decrease the effective duty of feed-effluent heat exchangers.
• Like Chloride, organic bromide will convert to hydrogen bromide after hydrotreating and then react with ammonia to form ammonia bromide salt in the exchanger.
• Ammonium bisulphide (NH4HS) hydroprocessing reactors convert sulphur and nitrogen compounds in the feed to H2S and NH3. On cooling, these two compounds react to form NH4HS. In the absence of water, NH4HS deposits to form a crystalline solid that can cause plugging of the reactor effluent air cooler. This will occur at relatively low temperatures, 10-30°C. If the dew point of water is reached in the effluent air cooler (or water cooler) or if insufficient wash water is injected.
In summary, foulants are typically found on the feed side of the preheat exchangers include various gums or polymers, iron sulfide, and salts. The organic fouling due to gums and polymers results from the polymerization of unstable species in the unit feed. Therefore, in order to determine the risk of organic fouling for a particular feed stream, detailed analysis of the feed is required to determine the problematic species in order to evaluate the fouling propensity and mitigation strategies. Another key factor to consider is the oxygen content of the feed stream as this can promote the polymerization of various unstable compounds, particularly olefins. Therefore, it is a good practice to exclude oxygen from feed storage tanks using a nitrogen blanket. However, this method is ineffective with streams already exposed to oxygen. The inorganic fouling is mainly caused as a result of iron sulfide that can either be carried from upstream units or generated in-situ in the preheat exchanger network. Identifications of the contaminants source and mitigations are key to eliminate the inorganic foulants.
SERGIO ROBLEDO (Haldor Topsoe, Inc.)
To answer this question, we need to differentiate between feed-side fouling and effluent-side fouling. Potential causes and mitigations will depend on which side is experiencing the fouling.
Feed-side fouling in your F/E exchangers can be the result of:
• Olefins
• Oxygen
• Particulates
Olefins/Oxygen
Olefins are normally introduced with cracked stocks in the feed. Typically, olefin gumming happens at lower temperatures (300 – 350 °F). Gums formed from peroxides, as a results of oxygen contamination of straight run feed, usually occurs at >400 °F.
In the case of coker naphtha, conjugated diolefins are present which are highly reactive species. In the presence of very small amounts of oxygen, or at elevated temperatures above 450 °F, these molecules will radically polymerize to form gum that can foul exchangers causing poor heat transfer as well as high pressure drop. If the feed contains significant quantity of coker naphtha then these Diolefins must be removed to prevent gum formation.
The coker naphtha should preferentially be sent to directly from the coking unit to the hydrotreater to prevent contamination with oxygen. Even straight run stock, which may be part of the feed component, must be prevented from contacting oxygen by storing the feed in a nitrogen blanketed storage tank.
Even with strict adherence to avoid feed contact with oxygen, the diolefins in the coker naphtha can polymerize at elevated temperatures. A dedicated saturation reactor operating in the range of 300 °F to 450 F will ensure that these highly reactive species are removed from the feed before polymerization can take place. Once the diolefins are removed from the feed then the feed can be heated to the required temperature for the required operating scenario.
Keep in mind that even though cracked stocks are not fed directly to a unit, there is potential of introducing cracked stock in sites that process slop in their Crude unit.
Particulates
Particulates, at high enough concentrations, in conjunction with low tube velocities, can result in these particulates settling out and plating on the surface. If no filter is present, then plans should be made to engineer and install a filter system to reduce the amount of particulates present in the feed. There are also companies that offer tube inserts to reduce the likelihood of particulates settling out and plating on the tube surface, preventing a loss of heat transfer.
Even with filters and tube inserts, if any gumming is taking place in the exchangers, then any small amount of particulates present will be picked up by the gums formed.
Examples in industry where fouling occurred on the feed side are:
• Cracked stocks blended with Canadian crude coming down the pipeline. These formed gums with oxygen and fouled the exchangers.
• Crudes from Venezuela blended with cracked stocks.
• Virgin jet was exposed to oxygen and fouled in exchangers operating >400 °F (this happened in multiple units).
o Similar example with natural gasoline.
As mentioned before, preventing oxygen ingress via direct, hot feed of cracked stocks to the unit, along with floating roof and/or nitrogen blanket on tanks is imperative. Tube inserts are also a viable option to prevent fouling where tube velocities are low enough allowing particulates to drop out in the exchanger. Most importantly, quality control is paramount in preventing this, or reducing further loss in performance. Examples of actions are:
• Notify the shipper and have the diluent stream changed.
− What is the crude source? Are there potential cracked stocks coming down the same line? How about Canadian crudes?
• Notify crude supplier about the poor quality.
• Install an oxygen stripper.
− Done for the virgin jet example.
• Install nitrogen blanket on feed tank or change to floating roof tank.
• Inject antioxidants into the tanks (mixed results).
• Bypass tank and go hot (direct) to the unit.
As for effluent side fouling, this is typically the result of NH3Cl (salts), which are the result of high levels of Cl in the feed. A water wash should be installed to remove these salts, and continuous is recommended versus intermittent.
• A licensor can help calculate where the water should be injected.
– Need to inject enough water and at the right spot to keep it as a liquid and not vaporize
– Liquid water will wash out the salts while steam will not
– Licensor can calculate where the dry point will occur and how much water needs to be injected
• Many examples of where water wash helps
– If done at the right spot with the correct amount of water and at the right temperature
• Boiler fouling
– Water treatment company can help with this
There is also a very good P&P this year covering reactor effluent diseases jointly presented by Flint Hills Resources and Marathon. Please plan to attend to learn more.
Question 36: What are your primary indicators that a coker furnace spall is complete? What steps do you take to optimize the efficiency of spalling?
GAMBOA-ARIZPE (CITGO Refining & Chemicals, L.P.)
A cautionary foreword: Online spalling of furnace heater tubes is not suitable for every heater design. It is necessary to consult with your furnace licensor or manufacturer to determine if online spalling practices are compatible with the heater designs installed in your respective facilities. There will be a more complete answer in the final transcript, including a more detailed discussion on the practice of delayed coker furnace spalling and the factors used to determine when the decoking operation of the furnace is needed. The verbal answer will only address the descriptive metrics currently being employed to determine when a furnace spall is considered ‘complete’. Technically, however, the primary question here is a difficult one to answer, because the completeness of a spall can only be determined post-spall with the furnace back online and after tube wall temperatures are measured; and to a lesser degree, after the heater pass pressure drop on normal oil flow has also been measured. Fortunately, the completeness of a spall can also be based on previous experience with a particular furnace and can be managed procedurally. That is the basis on which I will attempt to answer this question.
CITGO Petroleum Corporation operates four delayed coker units amongst its three U.S.-based refineries. Three out of the four units incorporate routine spalls for their respective coker furnaces as part of a broader operating strategy to optimize effectiveness of their overall furnace operation. The fourth delayed coker unit relies primarily on ‘piggings’. The benefits of online spalls versus steam/air decokes versus piggings will vary depending on the logistics of a facility, as well as on the heater’s mechanical configuration and its design. The decoking effectiveness of the three methods is also different. All methods, however, fundamentally aim to remove as much of the fouling coke layer that builds up along the interior surface of the furnace tubes as is possible during the operation.
The buildup of coke within coker furnace tubes is intrinsic to the delayed coking process due to the nature of vacuum residue material once it becomes exposed to the relatively high temperatures that are required by the process (greater than 850°F). While the buildup of coke cannot be totally averted, the rate of this buildup can be managed, depending on the heater design and other factors. The thickness of the accumulated coke layer can be generally inferred by monitoring and trending the furnace tube wall temperatures. Higher tube wall temperatures are indicative of thicker coke buildup because the deposited coke layer has lower thermal conductivity than the metal substrate of the furnace tubes. In effect, the coke layer acts as an insulating barrier that restricts the transfer of heat from the fire box into the process stream, which then allows the metal temperature of the tubes to increase.
Over time, the tube wall temperatures increase as the coke layer thickens. The sections of the heater that begin to experience faster coke buildup also shift with time as more and more tubes begin to experience lower heat transfer. This dynamic is caused by the need to keep the target coil outlet temperature (COT) relatively constant to maintain viable C5+ liquid yields and because the total heat flux across the heater [for a fixed surface area and a known dT (delta T; ∆T; temperature differential)] is also relatively constant. In other words, the gradual reduction in heat transfer efficiency across some of the process tubes forces the furnace to be fired at increasingly higher rates. This firing shift increases the localized heat fluxes in other sections of the heater to meet the specific COT that is dictated by the operation.
Removal of the coke layer at some routine maintenance interval improves the furnace’s overall heat transfer efficiency because it eliminates the insulative barrier that prevents the heater from meeting those COT targets at lower firing rates. Because of this dynamic, the tube wall temperatures tend to increase logarithmically over the course of a run until the coke layer is again removed. The run-length of this recurring operation must be optimized to maximize the refinery’s profits while ensuring that the long-term reliability of the equipment is not compromised.
Ultimately, the delayed coker furnace is decoked on an as-needed basis to prevent operation that could produce tube wall temperatures above the limiting design metal temperature of the respective alloy of the furnace tubes [as defined by API 530 (Table 5) or an equivalent table (ANSI, ASME, etc.); see my second slide entitled “Table of Limiting Design Metal Temperatures for Various Heater-Tube Alloys”]. The timing of a decoking operation is, therefore, dictated by the need to manage the reliability of the equipment, particularly to avoid encroaching the mechanical limits of the furnace tubes themselves; especially, long-term operation at the elevated temperatures. Equally important, an alloy’s critical temperature should generally never be exceeded as this could result in changes to the alloy’s microstructure. Note: Per API 530, “Other considerations can require lower operating temperature limits, such as oxidation, graphitization, carburization, and hydrogen attack.”
The API 530 limiting design metal temperature is not necessarily the strict driver for the end-of-run (EOR) tube metal temperature, although one could say it is good practice not to exceed it. The real driver for the EOR temperature is an economic optimization question: If a refiner chooses too high of an EOR temperature, then he or she will have to retube the heater excessively/ often; and if the refiner chooses too low of an EOR temperature, then he will have to decoke excessively/frequently. The short-term mechanical integrity limit for a typical coker furnace tube is appreciably higher than the typical EOR temperature. The tube geometry is a factor as well. For a thick-walled tube, the stresses are lower so higher temperatures can be tolerated. At CITGO, the online spalling operation was introduced as a viable decoking practice as early as 2001, with conceptual preparation going as far back as 1999. The written procedures themselves have been optimized at each respective facility via post-spall performance-based evaluations. Trial-and-error adjustments, both to the duration of the overall spalling and to the variable knobs of key procedural steps, have also been modified and incorporated over the years to produce the current procedures being employed today. There is some procedural rigidity for certain steps, but experience has also resulted in producing procedures which allow for some flexibility in other steps. The success of the online spalling operation depends on the combination of various factors. But in a broader sense, four gates must be cleared for the spalls to adequately remove sufficient coke buildup and restore heater performance.
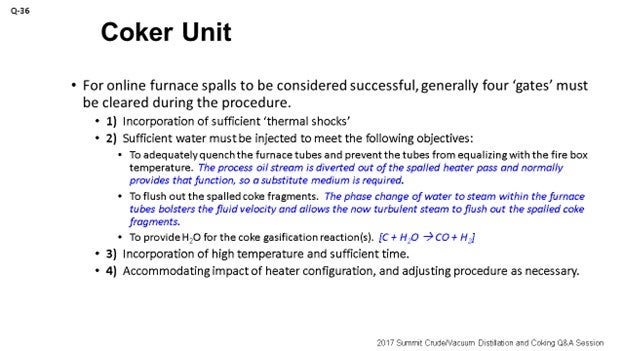
In a general sense, the term ‘spall’ describes the physical action in which chips or fragments are splintered and broken off of a larger solid body. There are several mechanisms that can produce a spall. In the context of the delayed coking process, however, the aim of furnace spalls is to break the coke layer off of the interior walls of the furnace tubes. This dynamic is produced by heating and contracting the furnace tubes in an alternating fashion over a relatively short time span to thermally shock and alter the fixed volume in the tubes themselves. Since the coefficients of thermal expansion of the metal substrate and the fouling coke layer are significantly dissimilar, the two layers grow and contract at different rates, which causes the physical detachment of the coke layer from the metal surface. The objective of thermal shock is to physically break the coke layer by using the force that is produced by thermal stresses to fracture the foulant layer. The first gate that must be satisfied in the spall, therefore, is to ensure that sufficient thermal shocks are incorporated during the procedure. Some are incorporated on the frontend of the spalling procedure, while others are incorporated on the backend of the spall.
During the spalls, boiler feedwater is lined up to the spalled heater pass, usually upstream of the convection section. Water addition serves three purposes, and I have them listed under the second bullet point in the slide. The three purposes are to:
-
Adequately quench the furnace tubes and prevent the tubes from equalizing with the fire box temperature. The process oil stream is diverted out of the spalled heater pass and normally provides that function, so a substitute medium is required.
-
Flush out the spalled coke fragments. The phase change of water to steam within the furnace tubes bolsters the fluid velocity and allows the now turbulent steam to flush out the spalled coke fragments.
-
Provide H2O for the coke gasification reactions.
The second gate that must be cleared is that sufficient water must be injected into the spalled heater pass to provide these three necessary functions. Water addition should be ratably controlled to target specific zones along the spalled heater pass and can be done by monitoring the progression of two wall temperatures in those specific sections over the course of the spall. Note: Boiler feed water or condensate is typically used as the source water because it needs to be free of inorganics/minerals (calcium, magnesium, sodium, etc.).
A combination of high temperature and time is needed to clear the third gate. The optimum value for both of these parameters must be determined through local experience while remaining within the temperature limits of the affected tube alloys. Procedurally, the time factor – or ‘length of hold’ step – should not be too rigid, given the fact that effective spalls can be performed to varying hold step lengths and dependent upon the morphology of the foulant coke, which can change and also be dependent on the feed slate. The combination of high temperatures and high steam velocities is required to micro-spall the coke layer via erosion and gasification reactions (where the steam can directly react with the coke to produce H2 and CO). The time factor simply provides a window for these two mechanisms to occur. The bulk of the coke removal during a spall operation may actually occur from micro-erosion and coke gasification, given the gradual change in tube skin temperatures that is typically observed during spalls.
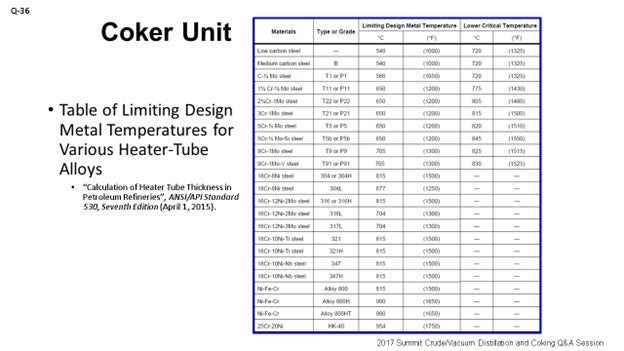
The fourth and final gate is more or less dependent on the heater design. Typically, a heater pass is spalled individually while other the heater passes remain on oil. This approach creates the possibility that the spalled heater pass may be affected by a neighboring sister pass. The fourth gate simply acknowledges the impact that a sister pass can have on the spalled heater pass and accounts for its heat input. Simply stated, a sister pass may need to have its respective coil outlet temperature target lowered prior to the introduction of oil into the spalled heater pass so that the combined firebox heat fluxes do not adversely affect the spalled heater pass in an acute fashion. Experience has taught CITGO that as much as half of the spalling benefit can be squandered from the onset if the effect of the sister passes is not taken into account. Along the same vein, another consideration to preserve the benefits of a spall prior to the conclusion of the spalling operation – and once oil is reintroduced into the pass – is to ensure that the core outlet temperature targets for the spalled heater pass be ramped up slowly. This precaution is needed to prevent higher flux conditions during the period when the oil flow in the spalled pass is not yet sufficiently high to properly quench the tubes.
It should be noted that spalling operations are not as effective on the process convection tubes, because even the minimum water addition requirements may over-quench and prevent the convection tubes from getting hot enough for the spalling mechanisms to work as effectively. Fortunately, the process convection tubes generally do not coke up as severely or as rapidly as the furnace’s radiant tubes. Eventually, however, the loss of heat transfer along the process convection section can become limiting enough and impose a higher duty load on the radiant section (leading to more accelerated heater pass coking rates in that section). Because of this ‘diminishing return’ dynamic, a heater that is normally decoked with online spalls will also need to incorporate a steam/air decoke or a pigging operation – roughly after every three or four spalls – to better restore the performance of the convection section.
In summary, sometimes short spalls are successful; sometimes they are not. Sometimes spalls with less water injection are successful; sometimes they are not. Sometimes spalls at higher temperatures are successful; sometimes not. Generally, however, if furnace tube wall temperatures can be uniformly reduced by 150 to 200°F post-spall, then the spalling operation can be deemed successful. This result will typically occur if all four gates discussed above are satisfied. Of course, there are a myriad of other factors one must consider with the spalling operation, such as effects on coke morphology, the coke cutting operation, some additional reliability considerations, and effects on the heater pass outlet manifold. These other factors usually do not affect the performance of the spall itself; they just need to be considered.
LÉGARÉ (Andeavor Martinez Refinery)
I will try to fill in some of the very few gaps that Héctor had in his talk. So, why is spalling efficiency important? If you are looking at a refinery where you are coker-limited, then coker spalling is essentially slowing down your whole refinery. It is really important that your Coker Operations team and your coker unit engineers are keeping a close eye on spalling to make sure that it is as efficient as it needs to be because it is really integrated into your planning process.
We will not go into why you do spalls, as that was already covered. Spalls really should be coordinated with the Planning Department. As I said, it is built into your plan, which is really managed by Operations because they will be the ones who will go through the procedural steps. Then, the unit engineer will be tracking the actual performance or the efficiency of the spall, which will be tracked as part of the unit health monitoring reports. As Héctor said, you cannot really tell how effective your spall was until it is done; so, online effectiveness is really just a myth and something that you should tell your manager that you cannot do. The effectiveness is really measured as a function of the delta T (∆T; temperature differential). That delta T is defined as the temperature difference between the post-spall start-of-run tube skin temperatures as compared to a baseline, which would be coming out of a turnaround or a physical pig. You really want to start to look at minimizing that delta T post spall because that will be the sign of true efficiency and effectiveness of the spall.
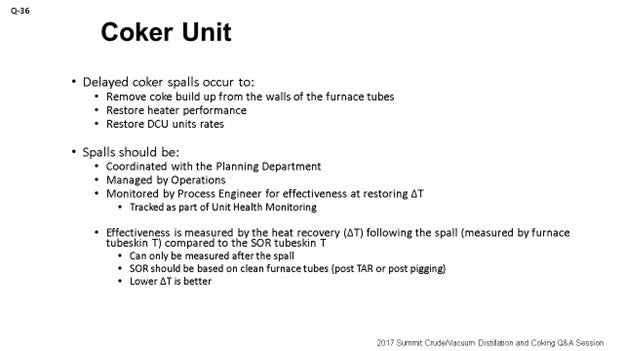
What can drive the efficiency of the spall? Héctor covered some of them factors. Obviously, one is the duration of the spall. Again, when you are in a planning situation and coker-limited, you will get a lot of pressure to minimize the spall duration. However, sometimes you will need to perform a longer spall. So, it is really important to keep that conversation fresh, and be upfront with the Planning Department.
The quantity and quality of the velocity steam you are using: Andeavor uses velocity steam, not boiler feedwater.
The next point is using a shock or continuous spall. The temperature of the spall will be temperature-dependent. So, with the shock spall, you will see temperature gradients playing a greater role as a continuous spall is more about velocity steam and constant temperatures.
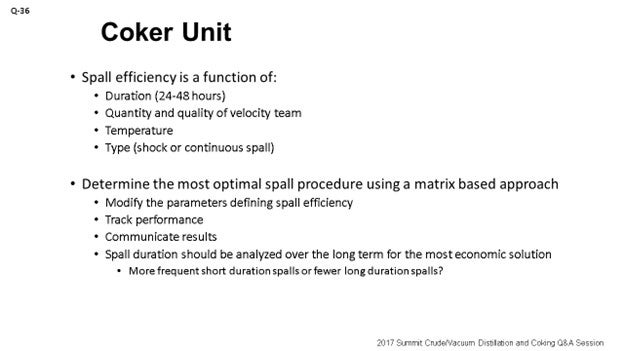
What can you do to optimize the spall approach? We came up with a chart, which you can see on the slide. The chart uses colors to measure performance. What do the colors tell us? Well, green means go; red means stop. The more green you see, the more delta T is being minimized and getting closer to zero. Red on the chart indicates that the spall did not go well. The point I want to make is that when a spall does not go well, you want to get with Planning right away and try to schedule another spall in that same pass as soon as you can. Because if you do not go after that pass right away, you may end up in a pigging situation. Like I said, green means it is directionally where we want to go. You can see there are different colors on the fonts, too. As we change the type of spall, we change the color of the font we use to represent the different spall durations utilized for the spall. So, the green and the red are the measures of the true effectiveness of the spall.
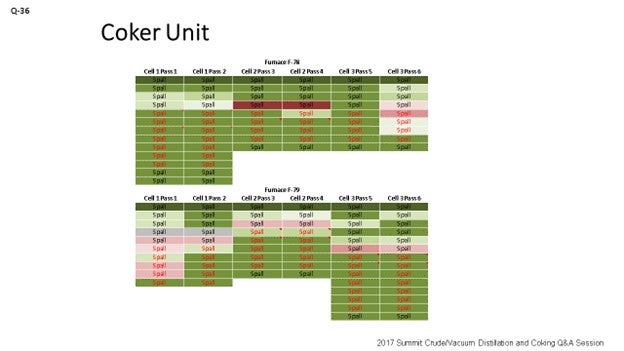
Some final thoughts: Optimized spalls are obviously going to help reduce lost profit opportunity; because when the efficient spalls are planned properly, they will be effective in getting the furnace performance back where it needs to be. Opportunity spalls are something that a refiner should really have in the forefront when looking at coker operations. ‘Opportunity spalls’ are the term we came up with when an opportunity essentially presents itself. For instance, you have some other upset in your refinery that has slowed down your coker. You have room on the coker rate, so you can go after a free spall essentially as long as you have the steam available to do that. Get your heater performance back up on track so that when you are able to push the coker again, you will not have to worry about that spall that was scheduled a week or two out.
I will tell you that the opportunity spall is something which needs to be ingrained in your culture; because as I have seen changes in the Operations team over time, they have lost sight of that opportunity. As a result, we have gone through an LPO (Lost Profit Opportunity) situation where we have had to cut back on crude runs only to come out and start the coker rates up again. And then within a week after raising coker rates, we had to slow down crude and coker rates again to spall. So, obviously, you want to try to avoid that type of coker slowdown. The last point is that the poor spalls really need to be addressed quickly. If you are not effective with a spall, you run the risk of going into a pig and having a shutdown.
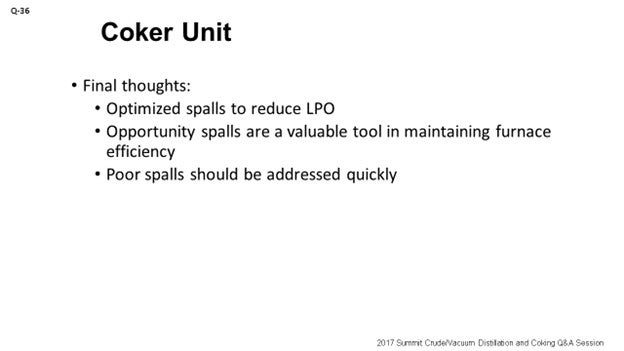
LOGEROT (Prosys Inc.)
You have heard the first two guys talk about trying to develop what might be considered an optimum procedure for the spalling. Héctor spent a lot of time on the four gates. So, I am going to introduce the concept from process control. Once you have decided on your optimum procedure, how will you be sure that you will follow that optimal procedure every time? There is a mechanism that, in the process control world, we call ‘procedure automation’. You can leverage your automation tools. They will allow you to automate your spalling procedure. So, ultimately, you get to a point where the operator just presses a button and says, “Spall this pass,” and the control system goes back doing all the spalling.
Now what I heard these guys say, too, is that some of the steps are rigid and that you want it to be on exactly this flow rate for this time and that temperature. Some of them are more flexible. You can build the flexibility into your procedure automation system by allowing the operator ranges of set points or controls for a particular part or step of the operation. So, basically, if you can write down your procedures in a stepwise fashion and provide the operating conditions that you want in each step, then it can be automated. When you automate it, you basically ensure that your “Best Operator” is on board all the time. Your “Best Operator” is really that control system which is controlling it and telling you exactly which steps you want and in what order, as well as which control settings to use each time.
ROGER METZLER (Baker Hughes, a GE Company)
When you are performing repetitive spalls, do you see a point at which you begin consistently getting diminishing returns and you just know you are only going to be able to perform so many spalls before you will have to set up a pigging or a decoke?
GAMBOA-ARIZPE (CITGO Refining & Chemicals, L.P.)
After several decoking operations, the refiner gets to the point where the spalls are no longer as effective. Usually, the convection section of the coker furnace becomes limiting because the spall is not as effective at cleaning the convection section. So, over the course of three or four spalls, you may have to come back and do either a steam/air decoke or a pigging operation to restore the performance of the convection section. Fortunately, the convection section does not foul as severely. It fouls a bit slower. But because of the general inability to get the right temperatures in the convection section tubes during spalls, the spalls are not as effective in that section.
One of the other points I want to make is that if you do have a spalled pass that did not perform as well post-spall – say you have a heater with four passes and one of them did not do so well – and if you do not do what Eric said and go at it again and reestablish a better spall, then you will end up with an imbalance on the heater. That imbalance is what will drive the heater to foul up more severely during the next run.
TARIQ MALIK (CITGO Petroleum Corporation)
I heard various times for the online spall. Darwin had it at 16 to 24 hours, and I think Eric said 24 to 36 hours?
LÉGARÉ (Andeavor Martinez Refinery)
Forty-eight.
TARIQ MALIK (CITGO Petroleum Corporation)
Forty-eight. So, what tells you that you are done? I would like to be done in 16 hours, but I can never complete a spall in 16 hours.
LÉGARÉ (Andeavor Martinez Refinery)
The range I gave will be in the Answer Book section of the transcript. We were basing it on a 48-hour spall because that was what we needed to get the performance we targeted. What happened was that we did not have the right information from our Inspection Department. As far as the temperature limits, we could see limits on the heater and the outlet piping temperatures. So, once we established a higher allowable outlet temperature on the furnace outlet piping, we were able to spall at a higher temperature and get the performance we needed in 24 hours instead of 48.
TARIQ MALIK (CITGO Petroleum Corporation)
May I ask at what temperature?
LÉGARÉ (Andeavor Martinez Refinery)
The revised temperature was 1300°F.
TARIQ MALIK (CITGO Petroleum Corporation)
For the next question, I want to poll the panel. At what tube-metal temperatures do you trigger the decoke, spall, or pigging of the heater? Do you go to 1300, 1400, or 1275°F? What is that number you reach where you say, “This is the limiting temperature; now decoke the heater tube”?
GAMBOA-ARIZPE (CITGO Refining & Chemicals, L.P.)
I think ours is between 1300°F and 1350°F.
TARIQ MALIK (CITGO Petroleum Corporation)
Is that 9-chrome tubes or stainless?
LÉGARÉ (Andeavor Martinez Refinery)
We are in the same range of 1300°F.
TARIQ MALIK (CITGO Petroleum Corporation)
Thirteen hundred? I do have one more question for Jeremy on that heater that does 12°F fouling a day.
THEISS (Marathon Petroleum Corporation)
I said 6 to 12°F.
TARIQ MALIK (CITGO Petroleum Corporation)
Okay: 6 to 12°F. I just took the upper number. You said that there are three cells. How many passes per cell?
THEISS (Marathon Petroleum Corporation)
Two.
TARIQ MALIK (CITGO Petroleum Corporation)
Okay. Thank you.
ERIC LÉGARÉ (Andeavor Martinez Refinery)
Delayed coker furnace spalls are performed to remove the buildup of coke on the inner walls of the furnace tubes in order to improve furnace heat transfer and maintain unit throughput and efficiency. As furnace spalls require coker and sometimes refinery crude rate reductions, they should be planned and communicated effectively to the refinery’s Planning Department to ensure that crude and product inventories are managed appropriately. Effectiveness of the spall cannot be measured during the spall, so it is only after completion of the spall that effectiveness can be determined. The Coker Operations Team will manage the spalling procedure and communicate spalling results for each planned spall. Spall effectiveness should be captured as part of the unit’s health monitoring report and tracked on an ongoing basis.
Spall effectiveness is monitored by the process engineer – after the spall is complete – by comparing the furnace tube skins’ start-of-run (SOR) temperature after the spall with a post-pigging or post-turnaround SOR furnace tube skin temperature. If post-spall SOR temperatures do not meet expectations, the details of the last spall should be investigated as changes may be required to the spall procedure and/or duration and will need to be communicated to Planning. A poor spall can introduce an unplanned event in the refinery’s monthly plan to recover coker furnace performance before the coke may prove too difficult to remove in a spall and pigging becomes the only solution. Note that the Coker Operations Team should always be looking for a window for an “opportunity” spall to regain heater performance when little or no refinery impact would be incurred.
Spall efficiency is optimized by maximizing spall temperature, which is measured as the furnace pass outlet temperature. Work with your Inspection Department to determine the true limits of your furnace tubes and/or furnace outlet piping. One Andeavor site worked with a lower furnace outlet limit for a couple of years. This resulted in longer spalling durations as effectiveness was impacted by the temperature limitations. The Inspection Department reviewed the piping metallurgy and provided relief on the historical spalling temperature limits.
Quantity and quality of velocity steam (high pressure, dry) is important when determining the optimal spall conditions. Determining the type of spall technique – shock spall or continuous spall – will also affect the efficiency of the spall. Shock spalls will utilize a cycling of spall temperatures to try to break off the coke using a mix of hotter and cooler temperatures in the tube passes. Continuous spalls will use constant temperature and velocity steam to help spall the coke off the tubes.
Spall duration can be seen as a tradeoff between the number and duration of spalls per year. If your spalling effectiveness is not meeting the expectations of your Refinery Leadership Team, it is recommended that a plan be developed using a test matrix to determine the most effective spall method for your unit. Higher spall temperatures within established limits and longer spall durations are preferred, but optimization to minimize durations will always be requested by your Planning Department.
Below is an example of a test matrix which can be used to develop an optimized spalling technique. An Andeavor site created a table that utilized conditional formatting to compare spall effectiveness and spall technique to generate a visual tool to demonstrate overall spall effectiveness. Darker green means spall effectiveness closer to baseline, with dark reds indicating a poor spall. Font color is used to explain the duration of each specific spall. Parameters that were changed included duration and furnace outlet temperature. Changes led to a new spall procedure that takes 24 hours to achieve the same performance that spalls of 48 hours did a year ago. This modification reduces the LPO associated with planned spalls by up to half.