Question 96: What are your experiences using SOx reduction additives formulated with lower rare earth content?
Ray Fletcher (Intercat)
Cerium oxide functions as an oxidant and oxygen carrier: the mixture of two oxidation states Ce(III) and Ce(IV) creates defect sites in the crystal structure where oxygen ions are missing (oxygen vacancies) – these get filled up in the regen and ceria acts as a kind of monatomic oxygen sponge. Monatomic oxygen is more reactive than O2 hence ceria catalyses oxidation reactions. Also mixing in the regen is effectively improved as oxygen is transported around the regen as the particles move around.
Most other oxidants don’t do this (e.g., Pt promotes oxidation when two molecules meet on its surface, it doesn’t sponge the oxygen). So, ceria does play a rather special role. Simply decreasing the amount of ceria works to some extent, but clearly a point will be reached where efficiency drops off.
Intercat has developed and commercialized SOx reduction additives containing 50% less cerium. What Intercat has done is to “extend” the functionality of ceria by proprietary methods to improve the overall oxidation activity of the additive thereby allowing the ceria content to be decreased at equivalent performance. At present, there are now over 28 users of this technology. In every application the lower concentration cerium additive has performed equal or slightly better than the standard SUPER SOXGETTER.
Further, Intercat is utilizing proprietary technology developed within its new owner, Johnson Matthey, for further enhancements in cerium dispersion together with new oxidation packages which will enable a 75% or greater cerium oxide reduction. These technologies include the careful construction of the physical structure of the microsphere, deployment of manufacturing technology which controls both the location and the local concentration of the cerium particles plus the addition of co-promoters to the additive. These techniques have made it possible to improve the overall oxidation activity of the additive thereby allowing the ceria content to be decreased while maintaining equivalent SOx removal performance. Two trials of this technology have been initiated and are being base loaded into two North American refineries now.
Intercat, as well as other additive suppliers, has developed rare earth free SOx reducing additive. These additives of course are lower in cost but generally require much higher concentrations in the circulating inventory. Depending on the composition of the additive this may lead to cracking dilution and possibly loss in product yield. However, it is recommended that refiners employing SOx reducing additive consider these technologies in addition to the high activity additives described moments ago.
Matthew Meyers (Western Refining)
Western Refining LLC has recently trialed several SOx reduction additives with lower levels of rare earth. The first was at half the typical rare earth levels. At 1% dosing, the result was a pickup factor of roughly 15. The second addition had zero rare earth and provided a pickup factor of roughly 5 at close to 3% dosing.
Eric Griesinger (Grace Davison Refining Technologies)
Grace Davison’s SOx reduction additives, formulated with lower rare earth content to lessen the impact of hyperinflationary costs associated with rare earth compounds, have gained wide acceptance. Within Grace’s portfolio of SOx additive products and its accounts, customers that were able to make a change to lower rare earth formulated SOx additives have done so. FCCU locations currently operating under EPA Consent Decree trial protocol have remained with the original formulation available at the start of their trial periods. Only two additional refineries are in the midst of evaluations between Grace’s Super DESOX® additive and Grace’s alternative products. Otherwise, all of Grace’s globally situated customers, existing and newly acquired, are utilizing SOx additives formulated with lower rare earth content. Grace offers three new SOx reduction additives: Super DESOX® OCI, Super DESOX® MCD, and Super DESOX® CeRO. Super DESOX® OCI, optimum cerium input; mitigates costs associated with rare earth compounds, while demonstrating on par pick-up-factor efficiency to Super DESOX® additive. Super DESOX® MCD, maximum cerium dispersion, further reduces rare earth cost exposure, yielding suitable and cost-effective balance between SOx transfer ability and slightly increased dosing rate. Additionally, Super DESOX® CeRO is formulated without rare earth compounds. All three of these new products build on the success of Grace’s Super DESOX® additive performance. These offerings provide refiners with a range flexible option, enabling a balance between rare earth inflationary exposures and dosing rates, to achieve SOx emission compliance.
Below is an example of a refiner that historically utilized Super DESOX® and then switched to Super DESOX® OCI. Observed is the ability of Super DESOX® OCI to continue controlling SOx emissions within limits, at comparable dosing rates as was the case with Super DESOX®. Utilizing Super DESOX® OCI over Super DESOX® can result in a SOx additive cost reduction roughly 35%.
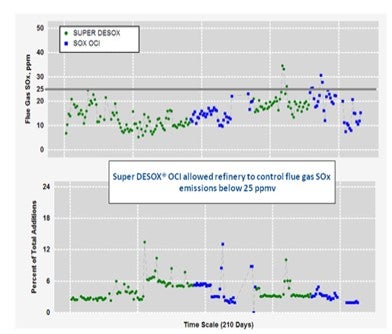
Additionally, Grace Davison’s laboratory scale research indicates that the partial burn environment performance of Super DESOX® OCI and Super DESOX® MCD is similar to that of Super DESOX®. Please contact your local Grace Davison sales and technical service representative for additional insight specific to your application.
Question 58: What issues are experienced at the desalter and pre-heat train when recirculating brine at the desalter?
SHENKLE (Flint Hills Resources, Ltd.)
Before answering this question, I want to clarify that the panel has defined ‘recirculating brine’ as brine going back to the freshwater makeup. For example, it may be used when insufficient makeup is available to maintain recommended washwater rates. We do not recirculate brine. We inject makeup water upstream of the second stage mix valve. Second-stage brine is pumped back to the first stage upstream of the mix valve, and then the first-stage brine is effluent. We operate washwater rates that are typically in the range of 4 to 5%.
SLOLEY (CH2M Hill)
Brine can be recirculated at the desalter. Additionally, there are some plants that recirculate brine found upstream in the heat train network. This is used in plants that have insufficient water to get proper contacting across the mix filter and which are often trying, in extreme cases, to move even from 2 or 3% water up to around 4 or 5% water. Since the freshwater rate does not increase when you do this operation, if it is more effective, you will increase the solid content of the brine. After all, that is the objective.
In some units, problems can arise due to oil and water emulsification because the pump that needs to recirculate this water – if you have oil in it – is a great mixing device. If the brine does not effectively de-oil, this water will recirculate and could cause problems with the rag layer in the desalter. Additionally, if the soap content of the water is high, you will get emulsions forming. With higher total water rates in many of these desalters also, the total water residence time is reduced, making the oil and water emulsions more difficult. The downstream exchange of equipment fouling and corrosion rate should be lower. If it is not changed or gotten worse, you should stop the brine recirculation.
HODGES (Athlon Solutions)
We are huge fans of recycling brine. In most cases, it is the Best Practice to increase the effectiveness of your desalter by increasing the effective washwater percentage through brine recycle, which will drive optimum desalting. As I mentioned earlier, one of the key items that is often overlooked when doing this is your seal flush. Make sure that you do not use the recycled water for your seal flush because it will erode your seal. Use fresh water. This may be subtle to some and obvious to others. Make sure that when you are recycling, you are not replacing your fresh water with recirculated brine. Recirculating brine is only used to add more effective percentage washwater. If you back out the freshwater, you will be taking a step back in effective desalting and contaminant removal across the desalter.
TOM COLLINS (Forum Energy Technologies)
Recirculating effluent water back to the desalter can improve efficiency by increasing water droplet population, allowing for larger droplets and faster settling. When recycle water is used, it is typically injected just before the mixing valve, not into the pre-heat train. It is also recommended that you divert the recycle when mud-washing unless a continuous mud-wash is used. Additional water volume may also allow for improved mixing efficiency, due to an increase in the water droplets created in the mixing valve or emulsification device. Care should be taken not to recycle water high in oily solids or other emulsifiers that may help stabilize interface emulsions and increase BS&W.
GLENN SCATTERGOOD (Nalco Champion Energy Services)
It is important to recognize the benefits of desalter washwater recycle, which improves dehydration and leads to improved salt removal. A higher rate of desalter washwater may also increase solids removal when processing high solids crudes.
DENNIS HAYNES (Nalco Champion Energy Services)
Recirculation of brine is a very good strategy to increase washwater to the desalter while minimizing effluent flow to wastewater treatment. The issues that may be experienced during this recirculating brine include a potential reduction in solids removal due to sending desalter effluent containing some solids through a pump motivating the flow back to the combined washwater inlet. More so, an issue is that if there is any upset or degree of oil in the effluent, the shearing action of the recycle pump will tighten the effluent emulsion. This emulsion, combined with the washwater into the raw crude oil which is then emulsified via the mix valve, may create interface growth in the desalter to the point that the system upsets. The brine recycle should be used with a non-oily effluent.
PHILIP THORNTHWAITE (Nalco Champion Energy Services)
It should be remembered that if a desalter operation is washwater-limited, the use of a brine recycle is an effective means of increasing the washwater volume and improving both dehydration and desalting performance. However, the operation is not without risk, and there are operation considerations to be made.
First, the recirculation of effluent brine is, in effect, adding salt to the crude oil when the two are mixed together. As a consequence of this combination, if the salinity of the brine significantly increases, the mixture can limit the salt removal efficiency across the desalters, the optimum salt content of the desalted crude increases, and the process efficiency can actually decrease. This reaction can be mitigated to an extent since the increased washwater volume leads to improved dehydration and desalting efficiency. Additionally, any increase in overhead chlorides can be mitigated to a degree through good monitoring and caustic management practices.
The other major consideration is that any deterioration in the effluent quality can have a significant impact on the whole desalter operation. If there is an upset leading to an oil undercarry, the oily brine will be passed through the brine recycle pump leading to the formation of a very stable emulsion. As this stable emulsion forms part of the total washwater feed, it can lead to emulsion layer growth within the desalter vessel and begin to exacerbate the already upset conditions. Key to mitigating this threat is regular visual checks of the try lines and effluent quality so that any onset in effluent deterioration can be quickly acted upon.
Question 34: What are your current protocols, practices, and concerns for using wireless communication between field instruments and the control room? Would wireless communication be acceptable for monitoring only, or is control allowed as well?
LOGEROT (Prosys Inc.)
The question asked about the protocols. But rather than naming the protocols, I think it would just be best to describe what the installation looks like. With wireless transmitters that are in use, you are usually installing a mesh network and you have a Modbus gateway connected to the DCS. You have a separate wireless gateway that is connected to that as well, and the wireless gateway connects to all the transmitters. Each one of the transmitters can actually act as a hub and is able to receive and transmit data with the other transmitters. That way, transmitters can find other transmitters close by and multiple pass-backs to the gateways. So, if a transmitter is out of service for any reason, the other transmitters will basically find pathways around it to get communication back to the wireless hubs.
Note that in this kind of arrangement, you need to have a robust gateway; because basically, the wireless gateway represents a single point of failure. Therefore, most installations use redundancy there, sometimes triple redundancy, to make sure that communication stays open.
Where are the main uses of wireless in refineries today? In our experience, they are usually remote areas of the refinery where a signal and power wiring are not easily run out. The big advantages, obviously, are cost savings, conduit wiring, and cable trays. Inside the battery limits of, say, a crude unit, wireless is not as common. Where used, it is usually for auxiliary type of measurements such as corrosion monitors, vibration monitors, additional temperatures, and pressures that are not central to the process. You might also consider wireless technology, as sometimes we have outside operators who have handheld devices that are wirelessly connected back to the control room. For that one-ring device, there will also be a network of gateways available for the wireless device to communicate back to the control room.
What are the concerns associated with wireless technology? The first real concern is cybersecurity. I mean, everyone is concerned about security these days. Basically, every wireless device and transmitter in your plant represents a potential entry point for intruders. So, you have to be very careful to put in strong security protocols to make sure that intruders will not get into your network. What can happen from an attacker? An attacker can jam your signals. You could lose proprietary data, and – worst of all – an attacker could end up gaining control over part of your process. You really do not want that to happen, which is why security is a big concern when using wireless. Second is overall reliability. Basically, we have been using hardwired signals for decades. Wireless signals are just not as robust in today’s technology as are the hardwired signals. For example, how often do you have to go reset the Wi-Fi in your house? That is an example of when wireless is not as reliable as it could be.
The last part of the question had to do with whether wireless is acceptable for control or if it is just for monitoring purposes. When I say ‘control’, I am talking about closed-loop control where you have a wireless transmitter communicating to the control room and there is a control action. They then signal out to the final element, usually a valve. It is probably also wireless, but it might be hardwired; but there is at least some component in that closed-loop control that is wireless. Our typical answer is that it is just not used very often for closed-loop control, and it is usually not recommended. One of the problems is battery life, because the transmitters you are using in the field are battery-operated. If you have a very high refresh rate – like, typically, a five-second refresh rate, then your batteries will die too quickly. That is one reason why you would not want to be using wireless for controls. So, generally speaking, it is not recommended or used. However, that is not to say that wireless control will not, sometime in the future, be relatively common.
THEISS (Marathon Petroleum Corporation)
The chart on the slide is really the internal guidance we use at Marathon. You can see that for what we call Class 0 and Class 1, we do not allow wireless communication, which basically inputs to an SIS (safety instrumented system) or some control point that is detrimental to the process. An example of a detrimental control point would be an FCC (fluid catalytic cracking) reactor/regenerator pressure differential transmitter for which we would not allow wireless control.
There are a couple of applications in which we allow control. I do not think they are widely used within Marathon; but with some corporate guidance and corporate technologist approval, we can use them wireless for control. These would be considered Class 2. An example would be a tray tower control for a temperature.
As you move further down the chart to Class 3, you can see an example of where you have the overhead water boot. You have a remote signal that goes into the board, but it relies on the operator to go out and make the move to drain that water boot and start to pump or open up a valve. Class 4 and Class 5 are really for informational purposes. My example for a Class 4 would be a secondary alarm on a tank where you have a primary alarm that is hardwired in and a secondary level or a backup level that could be used remotely. Class 5 would be temperature indication on heat exchangers just to gather data for fouling.
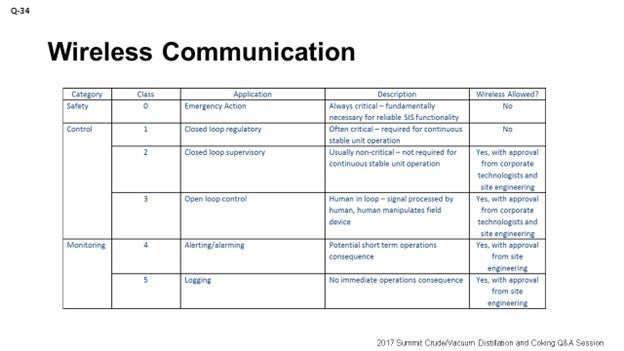
JEREMY THEISS (Marathon Petroleum Corporation)
Technology continues to progress in this field. Since 2011, we have had guidance that allows some usage of wireless instrumentation, but this technology is limited based on application. The table below identifies our stance on certain applications.
CATEGORY |
CLASS |
APPLICATION |
DESCRIPTION |
WIRELESS ALLOWED? |
Safety |
0 |
Emergency Action |
Always Critical: fundamentally necessary for reliable SIS functionality |
No |
Control |
1 |
Closed-loop regulatory |
Often Critical: required for continuous stable unit operation |
No |
2 |
Closed-loop supervisory |
Usually Non-Critical: not required for continuous stable unit operation |
Yes, with approval from corporate technologists and site engineering |
|
3 |
Open-loop control |
Human in Loop: Signal processed by human, human manipulates field device |
Yes, with approval from corporate technologists and site engineering |
|
Monitoring |
4 |
Alerting/alarming |
Potential short-term operations consequence |
Yes, with approval from site engineering |
5 |
Logging |
No immediate operations consequence |
Yes, with approval from site engineering |
Examples:
Class 0: Inputs to a Safety Instrumented System
Class 1: FCC Reactor/Regenerator pressure differential transmitter (used to manipulate flue gas stack valve)
Class 2: Tower tray temperature
Class 3: Water boot high/low level where control or field operator starts/stops a pump or opens/closes valve
Class 4: Storage tank secondary level alarm
In most of the approved applications, redundant wireless gateways are required to minimize disruptions to a failed gateway. Other points to consider for determining if wireless is acceptable include the required scan rate of the application, wireless distance limitations, and potential for wireless interference. Guidelines should be made to ensure battery life or alternate power to the wireless device is sustained and has monitoring capabilities.
DARWIN LOGEROT (ProSys Inc.)
Wireless Protocols
Rather than naming the protocols in use, it is probably better to describe the installation. Where wireless transmitters are in use, they are often installed in a mesh network similar to cellular towers or a Wi-Fi network with multiple hubs. A Modbus gateway is connected to the DCS and to the wireless gateway that is connected to all the transmitters. Each wireless transmitter acts as an individual hub and is able to receive and transmit data with others. This way, a transmitter can find other transmitters close by and have multiple paths to the wireless gateway. If one or two transmitters are out of service, the remainder will adjust to provide continuous communication.
In this arrangement, a robust wireless gateway is important. If only one wireless gateway is provided, it can represent a single point of failure, potentially losing view of all instruments using that path to the DCS. Users will typically install redundant gateways to mitigate this.
So, where are the main uses of wireless technologies in refineries today? The locations are usually remote where the signal and power wiring are not readily available. The big advantage is cost (savings in conduit, wiring, cable trays, power distribution, etc.) and the ability to monitor remote data, such as in a large, spread-out tank farm.
Inside a refinery process battery limit, use of wireless is not so common. Where it is used, some of the more common wireless applications are in corrosion monitors, vibration monitors, and additional temperature and pressure monitoring on vessels and exchangers (auxiliary to the hardwired temperatures and pressures).
Another use of wireless technology is for hand-held devices used by field operators. With this arrangement, another mesh network is employed to connect the wandering device to the DCS. The field operator can use the device to monitor operating conditions, execute periodic rounds, and take notes regarding observations. Major DCS manufacturers are offering this technology as an extension of the control system, but the control itself is done with hardwiring; only monitoring and setpoint adjustment are done through wireless.
What are the concerns associated with wireless technology?
First and foremost is cyber security. Every wireless device represents a potential entry point for an intruder. Security protocols are better and stronger now than ever, but many potential users are still reluctant to install extensive wireless devices. Security concerns include the possibility of wireless signals being jammed by an attacker, potential loss of proprietary data, or, worst of all, an outside intruder gaining control of part of a process.
The second concern is reliability. Wireless communication generally is less robust than hard-wired connections.
A third concern is the data refresh rate and its connection to battery life. For example, a one-minute update rate on the transmitters was tied to a life of about 10 years, whereas an update rate of four seconds reduced that life to two years. The relationship between refresh rate and battery life, of course, impacts how wireless can be used for basic control and impacts wireless maintenance costs.
So, is wireless communication acceptable for process control?
Allowing for a slightly wider definition of “wireless control”, it is in widespread use today – the plant radio. For example, the console operator can contact the outside operator: “Hey, go open/close the bypass valve around the control valve that is not working.” But more seriously, purely wireless communication for process control in a refinery is seldom used or recommended, especially in a unit that is tightly connected geographically, such as a crude unit or FCC. Where wireless communication is in use, it is almost exclusively for monitoring only, primarily due the problems outlined above. The closest approach to wireless control is using the handheld devices to adjust setpoints. The control itself is still through hardwire communications from the transmitters to DCS controllers and to the valves.
That said, there is no reason to expect that as technology improves, the current problems will not be overcome, at least in part. Perhaps future refineries will include widespread use of wireless process control.